
A Natural Combination
A three-in-one approach to drug delivery combines different actives in a hydrogel-based delivery method.
Polypharmacy – the concurrent use of more than one medication – is common in the elderly patient population, but complicated by strict instructions for use, which increases the risk of non-compliance. Oliver Lieleg, a professor of biomechanics at the Technical University of Munich, Germany, has been investigating how different APIs can be combined in a single delivery system that allows each drug to be released at different times. By combining hydrogels, artificial DNA and nanoparticles, he has shown that three APIs can be combined in an effective drug delivery system. Hydrogels are typically used in ointments, but Lieleg says that the concept could be applied to tablets. We spoke with him to find out more about the work.
What inspired your work?
Part of my inspiration for this project comes from an amazing publication from 2009; the researchers had developed a DNA-based nanobox that could be locked and opened again using single stranded DNA. Since then, I always wondered if DNA could be used as a tool in drug delivery. DNA base pairing is very specific and precise, and harnessing different levels of hybridization efficiency to allow for a controlled replacement of DNA strands by other DNA sequences seemed to me like a great way of engineering a sequential cascade of release events… And indeed it was!
I am quite amazed that, despite the progress we’ve made in the field of biomedical engineering and all the fascinating state-of-the art fabrication technologies available today, such as 3D printing, it still seems easier to make use of what nature offers and to find a different application for biological and naturally occurring materials rather than coming up with a fully synthetic system. Though it’s fair to say that nature has had much more time to optimize the materials it uses for controlling complex processes than humankind!
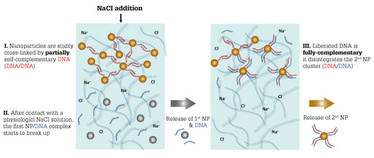
What are the main challenges in combining APIs and ensuring correctly timed release?
To my knowledge, releasing several pharmaceuticals from the same carrier is possible; however, most existing strategies try to retard the release process of a subset of those drugs (which basically entails different release kinetics) – but all release processes start at similar time points. Thus, small concentrations of all drugs will be made available at the same time, even if some APIs are not needed. Installing a logical control sequence into a drug reservoir, which only allows for the release of drug B once drug A has successfully released, is difficult. Nevertheless, that is the challenge we tackled and – at least for nanoparticles (which we use to model different drug carrier particles) – we were successful.
How does your DNA-based approach work?
We embed different clusters of nanoparticles into a hydrogel. In this aggregated form, the nanoparticles are trapped in the gel and cannot escape. However, if the clusters are broken up, individual nanoparticles can leave the gel by diffusion. We use DNA for two purposes: first, to create the nanoparticle clusters and, second, to break them up in a controlled, sequential fashion. By designing synthetic DNA strands such that they act as cross-linking, stabilizing agents on one cluster type, but induce disagreggation of another cluster type, we can obtain a controlled particle release sequence. In short, the break-up of the first cluster type sets free DNA strands which, in turn, trigger the disassembly of the second cluster type and so on. It is like opening a set of Matryoshka dolls – you only get access to the second doll once you have opened the first one. The first opening event gives access to the key needed to trigger the second opening event.
With our DNA-based approach, we can already create a successful release cascade of three different nanoparticle species from the same hydrogel. We can even design the system such that the particles located at the bottom of the gel exit first, and the ones closest to the surface exit last. Also, we showed that this DNA-based release mechanism acting on particles (for example, drug carriers) can be combined with other drug release control strategies acting on small molecules without causing unwanted interference. Thus, it is possible to control the release kinetics of molecules by classical methods (retarding their diffusive exit by employing binding interactions between the drugs and the hydrogel constituents) independently from the release of drug carrier objects (using our DNA-based strategy).
One of our next steps will be to apply a similar DNA-based control strategy to biopolymer-based nanoparticles that are actually loaded with drugs. Our hope is that we achieve a similar level of precision over the release process that we already obtained with inorganic, metallic nanoparticles so far.
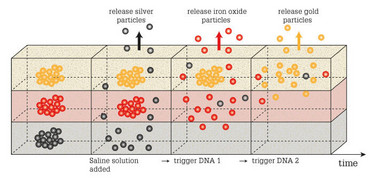
What consideration have you given to cost and increased scale?
At this point of our research, we have focused on the general feasibility of obtaining a sequential, ordered release cascade. I do not think that, at this time, the synthetic DNA sequences we employ are cheap enough to be used in a commercial product, such as an ointment or gel. However, with the field of DNA nanotechnology quickly evolving and with other groups from this field also tapping into application-oriented research areas, novel strategies are also being developed that aim to produce defined, artificial DNA strands at lower cost; for example, by making use of biotechnological methods and DNA amplification in microorganisms. With such developments, our mechanism might become affordable relatively soon.
What are the limitations of your approach?
At the moment, our strategy targets the release of different nanoparticles in a controlled, sequential fashion. Even though we have used metallic nanoparticles for our experiments, the DNA-based control mechanism can also be applied to other particles, such as liposomes or biopolymer-based nanoparticles – as long as their surface can be functionalized with DNA strands. However, this is a limitation of our approach, as it relies on such drug carrier objects. On the other hand, this also means that there are no specific requirements for the drugs themselves – they only need to be encapsulated into a certain type of drug carrier so that they can be integrated into our DNA-controlled release cascade.
What types of therapies would benefit from being combined?
I believe that our DNA-based release strategy might be most useful for regulating a time-separated release of different therapeutic agents from a hydrogel that is applied to a body location that is not easily accessible for self administration. I imagine that sensitive wounds, such as burns or internal wounds created after surgery, could benefit from such an approach. Here, it may be possible to release an antibiotic first and then, later, a drug stimulating cell proliferation. Moreover, with our mechanism, it is also possible to release three doses of the same drug, but at distinct, separated and well-defined time points. For this to happen, each drug carrier particle would need to be loaded with the same drug.

Making great scientific magazines isn’t just about delivering knowledge and high quality content; it’s also about packaging these in the right words to ensure that someone is truly inspired by a topic. My passion is ensuring that our authors’ expertise is presented as a seamless and enjoyable reading experience, whether in print, in digital or on social media. I’ve spent fourteen years writing and editing features for scientific and manufacturing publications, and in making this content engaging and accessible without sacrificing its scientific integrity. There is nothing better than a magazine with great content that feels great to read.