Reduce, Refine... Replace?
How to use organ-on-a-chip technologies to make drug discovery more efficient
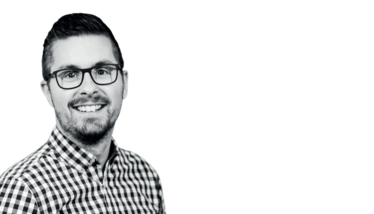
Why do so many drugs entering clinical trials fail? Too often, existing test models do not accurately predict human outcomes.
The rapid response to the COVID-19 pandemic has shown that safe and effective therapeutics can be developed and delivered to market efficiently by challenging – and improving – existing processes. We should now seriously examine other ways to improve existing drug discovery processes. Research suggests organizations could slash R&D costs by almost one third through the introduction of organ-on-a-chip (OOC) technology – alternatively known as microphysiological systems (MPS) – into their workflows, thus addressing the problem of human-relevance in traditional 2D cell cultures and animal models (1).
Adoption of MPS has risen over the last decade, but there are still misconceptions about their effective use and potential value. MPS are often framed as an eventual replacement for animal studies and, whilst there’s a way to go before this becomes a reality, recently the European Parliament resoundingly passed a resolution to phase out animal testing in research, regulatory testing and education, which will put more focus on the use of new alternative methods (NAMS) such as OOC. Others are concerned about the acceptability of MPS data in Investigational New Drug (IND) submissions. In the meantime, MPS are being used to drive major improvements in the accuracy and efficiency of drug discovery. Researchers should understand that MPSs represent a concrete approach to reduce, refine, and complement existing tests, right now – rather than a futuristic proposal to sweep away the status quo.
Using microfluidics, MPS devices generate in vitro 3D models that faithfully recapitulate the in vivo phenotype and function of equivalent human (or animal) organ counterparts. They differ from static culture approaches in their use of fluidic flow to mimic blood circulation. In doing so, these systems facilitate microtissue development, improve physiological relevance, promote culture longevity, and facilitate inter-organ communication. Most MPS technologies are high content rather than high throughput – they compare tens or hundreds of molecules against a known target or pathway to deliver deep mechanistic insights.
At the beginning of the drug discovery process, MPS can be used to complement the reproduction and corroboration of target-specific data from patient-derived clinical samples, test animals, or other preclinical tools. MPS simulate an in vitro state that expresses the same targets as a human disease, helping to identify the role and relevance of these targets. Critically, MPS cultures are more physiologically relevant and cheaper than animal models. These same predictive models can also be carried through to lead optimization to complement and improve animal (in vivo) efficacy studies. They could unlock options to explore many more drug concentrations in vitro, helping to refine the effective therapeutic dose range ahead of animal studies. Through cross-referencing the results, potential cross-species issues would be flagged early. In my view, MPS are especially beneficial for testing the safety and efficacy of new human-specific modalities where differences in genetics, metabolism, or immunological response render animal models unsuitable.
MPS are better predictors of human-specific adverse effects than the standard range of pre-clinical safety toxicity tests. Standard animal and in vitro models have proved poor predictors of drug-induced liver injury (DILI) – a major contributor to late-stage drug failures and withdrawal from the market – due to their non-human metabolic profiles. Liver MPS provide researchers with the means to uncover potential adverse metabolite-driven effects early, reducing the risk and cost of late-stage failures. Additionally, disease modeling with MPS can identify increased DILI susceptibilities for classes of patients with underlying metabolic conditions, such as the hugely prevalent condition of non-alcoholic fatty liver disease, reducing the risk that therapeutics exacerbate pre-existing conditions.
Above and beyond the benefits of single-organ models, researchers can now also link multiple-organ MPS systems together to represent the interaction and communication of complex human systems. At present only animal studies offer such insights, but interspecies differences can lead to costly, unforeseen late-stage problems. One clear multi-organ application is for human ADME profiling. By connecting a liver MPS to another “route of entry” organ (for example, gut or lung) to monitor drug transit through an interconnected system, MPS can provide researchers with a human in vitro alternative to animal or in silico first-pass metabolism studies for improved bioavailability determination. To increase the accuracy and precision of data translation, in vitro to in vivo clinical predictions can be extrapolated using physiologically based pharmacokinetics (PBPK) mathematical models.
By incorporating MPS into drug discovery and development at pivotal and strategic stages, we can cross-validate or otherwise supplement currently available datasets. Indeed, in combination with existing methodologies, MPS can highlight unexpected surprises before the clinic. For me, MPS represent an essential but complementary tool in the preparation and evaluation of IND data packages.
As these increasingly advanced in vitro models continue to prove their worth and take on new roles, we anticipate that there to be a natural “survival of the fittest” evolution. Unless parliamentary pressure accelerates this process, MPS will not suddenly replace or eliminate traditional approaches, but in the meantime, its complimentary use will provide a path to more insightful and efficient drug discovery.
- N Franzen et al., “Impact of organ-on-a-chip technology on pharmaceutical R&D costs.” Drug Discovery Today, 27, 1720. (2021). DOI: 10.1016/j.drudis.2019.06.003.
Chief Scientific Officer, CN Bio, Cambridge, UK