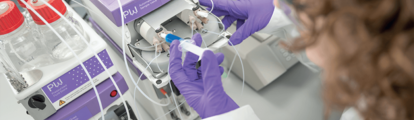
Lipid Nanoparticles: From Little League to the Majors
As lipid nanoparticles have evolved, the number of potential applications has grown to include not only small molecule drugs and vaccine delivery, but also gene therapies and more. But what does the future hold?
Bowen Tian | | 8 min read | Review
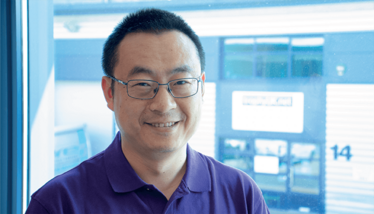
Image Credit: Particle Works
The COVID-19 pandemic brought lipid nanoparticles (LNPs) to the fore, creating a surge in interest as the pharma sector exploited their benefits for mRNA vaccine delivery. But they have been around for much longer. LNPs are based on well-established liposome technology developed after British scientist Alec D Bangham first discovered liposomes in the 1960s. LNPs are formed by adding ionizable or cationic lipids to liposomes to allow the encapsulation of negatively charged oligonucleotides – such as RNA and DNA – through electrostatic interactions. The lipid shell then serves to protect the encapsulated genetic material against premature degradation in vivo until it reaches the target cells.
After 30 years of effort, the FDA finally approved the first liposomal drug – Doxil® – in 1995. A few years later, surface conjugation of antibodies became a popular area of liposome research, as the large biomolecules were able to bind to specific receptors on cancer cells. However, antibody-targeted liposome delivery systems failed to improve therapeutic effect compared with Doxil in clinical trials. More recently, the first liposomal gene product for siRNA delivery, Onpattro®, was approved in 2018, making it possible to treat liver disease by intravenous administration of LNPs carrying therapeutic genetic material.
Liposomes and LNPs have many proven advantages as drug delivery systems, as they greatly reduce the likelihood of drug-associated cardiotoxicity – a key benefit Doxil is known for – and offer the ability to preferentially target the tissue of interest. We’ve already covered their unprecedented success in vaccine delivery; it’s widely known that several COVID-19 vaccines – such as those from Moderna and Pfizer-BioNTech – use LNPs to deliver mRNA into cells, where it is released to produce proteins that aid in counteracting the SARS-CoV-2 virus.
However, one of the most exciting areas for the future of LNPs is gene therapy, where they are proving their value as non-viral delivery vectors. Traditional viral vectors have a limited gene delivery capacity, typically fewer than 10 kilobase pairs (10,000 base pairs) and require a complicated engineering and manufacturing process. They also often stimulate an unwanted immune response within the body – for example, inflammation and organ failure – and have the potential to cause mutagenesis by inserting their own genes into the genome of the target cells. Non-viral vectors, such as LNPs, have emerged as a way of overcoming all of these problems, removing the limitation on the size of the gene you can deliver and reducing the risk of adverse effects. As work with LNPs continues to increase across the industry, there are two areas that warrant close consideration: production processes and drug delivery methods.
mRNA: the transient gene therapy
The current RNA-based therapies on the market principally control the symptoms of diseases, rather than permanently curing them. However, there have also been great strides in the development of permanent gene therapies over the last few years. For instance, CRISPR cas-9 is able to precisely delete a specific gene in vivo for the treatment of liver disease when injected intravenously. Based on this success, scientists are now going one step further, investigating the potential of making permanent insertions to replace injurious gene sequences.
At the same time, transient gene therapies are also gaining traction, mainly because permanent DNA insertions and deletions require extremely accurate gene editing, making them difficult to perform reliably, and so carry significant risks. mRNA-based therapies are an example of this approach, as they lead to the expression of proteins for just a few days, after which time the mRNA is cleared out of the body. This alleviates concerns regarding accumulation in the tissue or severe side effects in the long term.
Several biopharma companies, such as BioNTech, are now producing combination therapies that deliver cancer antigens using an LNP-mRNA vaccine. The antigens activate the body’s own immune system, stimulating it to produce antibodies and specific B and T cells against the cancerous cells. This results in the body recognizing and attacking the tumor itself in conjunction with checkpoint inhibitors, as well as better preparing it to recognize future cancerous mutations, minimizing the chance that the cancer will recur. However, cancer tumors also have their own defense system which allows them to avoid the attack of the immune system, and also induces T cell death. Therefore, for maximized therapeutic effect, it’s necessary to administer additional treatments to weaken such tumor dense system, in combination with the mRNA vaccine. These combination therapies could include a check point inhibitor, or even genetically engineered T cells, such as CAR-T cells, which are able to directly kill cancer cells.
The production problem
LNPs are formed through a self-assembly process that is very difficult to control and scale up, incurring substantial financial costs that can be unviable for many biotech companies. As a result, much effort has been poured into improving LNP-mRNA complex formation through more precise mixing of lipids and mRNA to increase the consistency of the process. An example of this is a widely applied ethanol injection method, where a syringe is used to inject lipids suspended in ethanol drop-by-drop into aqueous mRNA solutions. T-mixing is another common option, and is mostly used in large-scale LNP synthesis.
However, neither of these batch production methods can generate truly monodisperse LNP-mRNA nanoparticles, and they can lack batch-to-batch consistency in terms of particle size, composition, and morphology. Additionally, the shearing forces applied to the particles by these techniques could accelerate degradation and rupture the LNP-mRNA nanoparticles. The result is that most LNP-mRNA treatments are unnecessarily costly with low therapeutic efficiency. In fact, numerous studies have shown that less than four percent of LNP-mRNA nanoparticles are capable of effectively releasing mRNA intracellularly in cell culture, and this is believed to be even lower when administered into the muscle (1,2).
Microfluidic devices could provide advantages compared with ethanol injection and T-mixing, as they maintain consistent conditions for LNP self-assembly and offer greater process control. This approach makes it easier to determine the formulation that works best in terms of both maximizing therapeutic effects and minimizing side effects. Unlike traditional production methods, microfluidic systems rely on fluids flowing into a microfluidic chip from separate channels, meeting at the junction and being actively mixed in a reproducible manner to form nanoparticles. Depending on the fluidic chip design, this allows precise mixing of fluids down to single microliter volumes, and continuous operation, rather than batch production. In addition, an optimized microfluidic set-up generates highly monodisperse LNP-mRNA nanoparticles, with improved homogeneity across the population in terms of lipid composition, mRNA payload, and morphology (3). These particles also show greater mRNA loading efficiency, which has the dual benefits of reducing wastage of expensive materials, and potentially allowing significantly reduced doses.
On top of this, microfluidic workflows are well suited to automation and high-throughput manufacture, making it possible to produce more vaccine or therapeutic drug within a short space of time – for example, when under time pressure during a pandemic. Automated, high-throughput methodologies are also generally more cost-effective than batch techniques, which tend to be more labor and time intensive.
Ongoing developments in delivery
LNPs containing therapeutic genetic material or other APIs can be delivered to the target tissue either systemically or topically. Systemic delivery is typically achieved by intravenous administration, such as in the treatment of liver disease. Once the LNPs reach the organ of interest, they must penetrate the tissue and be taken upby the target cell type, for instance, through receptor-mediated endocytosis. The LNP then disintegrates, the therapeutic is released and, after endosomal escape, it begins acting on target pathways. At present, the mechanism of endosomal escape is not yet fully understood, and its efficiency is thought to be very low. The particles’ protein corona may have a large influence on the performance and capacity to target the tissue of interest, but the underlying mechanisms of this are still under investigation. These big unknowns have sparked a significant amount of research into how to design LNPs for optimal performance, ensuring efficient endosomal escape and preferential accumulation in the target cell type. There has also been a recent focus on LNP-based delivery mechanisms that are capable of crossing the blood-brain barrier.
Delivery can also be localized, being performed intramuscularly, transdermally or by inhalation using LNPs that have been aerosolized in a nebulizer. Inhalation is now one of the most widely pursued delivery route, with several clinical trials currently ongoing. This method delivers therapies directly to the lungs and can be used to treat severe diseases like cystic fibrosis, making it a particularly promising delivery technique. However, the biggest challenge for delivery by inhalation is that the vibrating mesh used in a nebulizer for aerosol formation may damage the LNP structure, causing early RNA degradation and loss of biological function prior to reaching the cells of interest. There is, therefore, an ongoing need for a specially designed nebulizer capable of aerosolizing LNPs without causing damage.
Much left to learn
The community of liposome and LNP researchers remained extremely small for many years, but, unsurprisingly, it has grown exponentially since the COVID-19 pandemic. LNPs are clearly an exciting field of research, and they are generating a great deal of interest across multiple industries thanks to their versatility. RNA therapeutics in particular are causing a rapid revolution in medicine – and the field of gene therapies is booming. Almost any disease you care to name can potentially be treated using RNA-based gene therapies, and all the necessary technologies are already available to enable delivery via LNPs. Although this is a very challenging niche, novel genomic medicines would provide significant clinical benefits for a range of conditions, and offer sought-after solutions that could address currently unmet clinical needs. In short, there has never been a better time than now to work on LNPs for gene therapy.
- M Maugeri et al., Nat Commun 10, 4333 (2019). DOI: 10.1038/s41467-019-12275-6
- J Gilleron et al., Nat. Biotechnol., 31, 638–646 (2013). DOI: 10.1038/nbt.2612
- SJ Shepherd et al., Biomaterials 274, 120826 (2021). DOI: 10.1016/j.biomaterials.2021.120826
Tian has over 15 years of research experience in the field of nanomedicine. He completed his PhD at University College London, focusing on the use of liposomes and lipid nanoparticles for targeted delivery of therapeutic molecules, including drugs and genes for cancer treatment. Since then, Bowen has been applying his nanomedicine expertise in cutting-edge research – ranging from protein engineering to gene and cell therapy – in both academia and industry.