Hunting for a Cure
The world’s first clinical trial for a Hunter syndrome gene therapy opens in Manchester
Jamie Irvine | | 5 min read | Interview
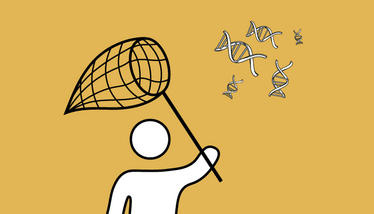
UK regulatory authorities have approved the first ever trial of a gene therapy for young children diagnosed with Hunter syndrome – also known as mucopolysaccharidosis type II (MPS II) – a rare lysosomal storage disorder characterized by short height, hepatosplenomegaly, and joint problems, among other symptoms. The trial, led by University of Manchester researchers, and a clinical team from Manchester University NHS Foundation Trust, includes five children under one year of age who may receive enzyme replacement therapy prior to the gene therapy. The investigators hope the gene therapy can eliminate the need for weekly enzyme replacement.
Brian Bigger, Professor of Advanced Therapeutics at the University of Manchester, developed the therapy over eight years and recently published a paper validating proof-of-concept outcomes in mice. Here, he tells us more.
What’s the story behind the therapy?
Our journey into hematopoietic stem cells (HSC) gene therapy started with Sanfilippo disease type A (MPS IIIA), a similarly devastating neurological condition. In collaboration with clinicians Ed Wraith and Rob Wynn, we developed an HSC gene therapy vector for this disease in 2010. This lentiviral vector targeted monocyte cells that traverse into the brain, employing a CD11b promoter, and was among the co-founding initiatives of Orchard Therapeutics in 2016. Currently, we are conducting a clinical trial for HSC gene therapy in Manchester for MPS IIIA, and working alongside Simon Jones (Wraith’s successor) and Rob Wynn to develop HSC therapy for MPS IIIB and AAV therapy for MPS IIIC.
During our HSC gene therapy work and the Sanfilippo clinical trial with our vector, we observed a notable discrepancy; enzyme levels in the periphery were 20–50 times the normal range, whereas in the brain, they were only 1–2 times normal levels. Recognizing this challenge, our initial approach involved using blood-brain barrier crossing peptides to augment the enzyme’s entry into the brain. Around 2015, it became evident that this strategy was gaining regulatory approval in the enzyme replacement therapy field. Consequently, we decided to explore this avenue genetically and our efforts in this direction proved to be successful.
How does the gene therapy work?
Hunter disease results from a defective IDS gene located on the X chromosome; this mutation disrupts the normal breakdown of long-chain sugars within the lysosomes of cells, leading to an accumulation throughout the body. The impact is felt in the bones, joints, and heart of affected boys, with approximately two-thirds of patients experiencing brain involvement, with symptoms such as hyperactivity and loss of developmental milestones. The severity varies across a spectrum, but a shortened life expectancy is typical.
In our approach, we mobilize blood stem cells into the patient’s bloodstream, draw a blood sample, and transduce the cells with a lentiviral vector to replace the faulty gene. After conditioning, we reintroduce the modified cells. Though the IDS enzyme is produced from blood cells throughout the body, it faces a challenge in reaching the brain independently. Although blood stem cells can traverse the blood-brain barrier to deliver the enzyme, only a small proportion accomplishes this, limiting the enzyme dose in the brain.
To enhance this process, we have taken an additional step by incorporating a blood-brain barrier crossing tag, specifically the ApoEII tag, to the IDS enzyme. This modification enhances the enzyme’s ability to cross into the brain more effectively, thus improving the overall therapeutic outcome.
What preclinical results have you seen?
We demonstrated the efficacy of a targeted gene therapy for the brain in MPS II mice using HSC and a specific lentiviral vector expressing IDS.ApoEII. We then compared this approach to a lentivirus expressing native IDS and a standard bone marrow transplant. Interestingly, results at both the 6-month and 12-16-month marks post-treatment achieved sustained IDS enzyme activity in the organs of MPS II mice treated with IDS.ApoEII. There was also continual clearance of long-chain sugar storage in the brain and peripheral organs, sustained correction of abnormal innate immune responses in the brain (astrocytosis, microgliosis, elevated cytokines), and complete rectification of hyperactive behavior and working memory defects observed in the mice. Though using lentiviral IDS alone (without the tag) proved insufficient to correct behavior, we noticed a conventional bone marrow transplant could not address brain enzyme levels or reduce long-chain sugar storage in the brain. Either way, despite the overexpression of IDS-ApoEII product, the therapy was well tolerated in mice.
What was the biggest turning point for the work?
It was amazing when our program was licensed to Avrobio in 2020, and we successfully negotiated clinical trial funding in Manchester, once again collaborating with Rob Wynn and Simon Jones. Encouraged by the success of our Sanfilippo A trial, we anticipated this to be a pivotal moment for clinical treatment. Shortly after we opened the trial, however, Avrobio withdrew its support. Fortunately, they left us with sufficient resources to reopen the trial for patients, and we have since gained Lifearc funding to bridge the financial gap to ensure we can treat all the patients initially planned for the trial.
What is the focus of the current trial – and what are the timelines?
Given the singular nature of this intervention, we have incorporated several efficacy endpoints into our study. These endpoints are designed to obtain IDS enzyme levels both in the brain and periphery, while also evaluating the potential clearance of storage materials.
The trial is currently open for enrollment, and we plan for a two-year follow-up for the five patients we recruit.
What will be the biggest challenges moving forward with this therapy?
The cost of goods for manufacturing and the very tight regulations are two huge bottlenecks to progress in this field. Year by year, regulatory constraints on the manufacturing of gene therapy vectors and cell transduction tighten, leading to a disproportionate increase in manufacturing expenses.
Though ensuring the safe production of medicines is crucial, the escalating regulatory demands and consequent prices have forced our clinical team to reject patients from other trials. The rejections could have life-threatening consequences for these individuals as the cost of manufacturing has become unsustainable.
My aim in moving to the University of Edinburgh is to find solutions in reducing the cost of manufacturing of gene therapy vectors. I also aspire to develop advanced therapy products applicable to a broader spectrum of genetic diseases.
Associate Editor, The Medicine Maker