Down to Earth
Aiming for technologies that can make medicines in space or on Mars can also help us with problems closer to home, such as challenges with cold chain and getting medicines to remote locations
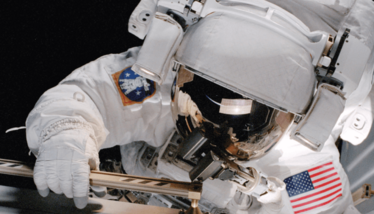
The excitement of working on projects that will enable human exploration of Mars is huge. I don’t think we’ll see people landing on Mars during my lifetime, but the greatest driver for me is knowing that if we can maintain and restore the health of explorers on Mars, then we can do it anywhere – in a hospital, on a nuclear submarine, in the Antarctic, or in a remote community in South America. Making a medicine on site and on demand, using resources that can be sourced locally – and ideally that are waste products from something else – would solve issues with cold chain. Cold chain supply accounts for roughly one percent of the total pharmaceutical market. Although one percent isn’t much, the numbers add up fast when you consider that the pharmaceutical market is worth well over US$1 trillion… and the vast majority of cold chain products (60 percent) are transported by air. If we can get rid of centralized manufacturing and make medicines for individuals where and when they need it, there will be huge advantages in terms of cost and the environment – as well as for patients, who will get the medicine they need tailored to them.
My group has done a lot of work with cell-free synthesis. From our work on in vitro evolution, we began exploring the use of synthetic biology to make proteins. Here, we take the machinery that a cell uses to make proteins out of the cell and put it in a test tube. With a DNA synthesizer, it is possible to make DNA strands of any sequence. We can give the DNA that encodes a particular protein to the cell machinery – which then produces the desired protein. Our cell-free protein synthesis method uses the machinery from an extremophile (Deinococcus radiodurans), combined with microfluidics and novel protein storage and reconstitution ideas. The project is funded by the UK’s Engineering and Physical Sciences Research Council (EPSRC), through their Adventurous Manufacturing program.
Once the protein is produced, it has to be formulated into a deliverable therapeutic. To do this, we are working with 3D printing experts. Some of my colleagues at the University of Nottingham – Clive Roberts’ group – are pioneers in additive manufacturing and in particular of pharmaceutical formulations. We are considering a variety of ways in which 3D printing can be used for formulation of the on-demand-manufactured drug, such inhaled drug delivery or dissolvable microneedles.
An on-demand approach to drug manufacturing could completely change how we make medicine – and realize the ultimate goal of telepharmacy, where a pharmacist or medical practitioner can transmit a particular drug and its formulation over any distance. Once the technology is in place to synthesize and formulate the drug on demand, all that is required is a set of instructions that tell the system how to make the drug (the DNA codes) and formulate the resulting protein into an appropriate dosage form (the printer instructions), with the correct release profile. We’re talking about a digital file that could be sent across continents… or across the solar system.
Theoretically, we could separate the drug discovery process from manufacturing and distribution. It would be possible for a drug discovery company to release a drug without having to go through the process of manufacturing and transporting it. They would just release the software or instructions for the drug, which could be downloaded across the globe to make the medicine. We’re not at that point yet, of course.
Biologics are considered the future of most medicines – and are the ones with the most demanding cold chain requirements – but there will also always be demand for small molecules too. We’re also looking at how to make small molecules the way nature does by using cell-free synthesis to make enzymes that can convert one material to another. It’s all early work and baby steps but, ultimately, we envision a system that can make protein therapeutics, and also proteins that can be used to make more molecules.
Spores for space
The project with Lynn at NASA and others builds on the cell-free synthesis method and uses spores, which have been shown to survive the environment of space outside of the ISS, to house the cell machinery. The idea is to create a system that allows astronauts to create biopharmaceuticals on demand. We are using a number of protein-based therapeutics as exemplars – a thrombolytic (stroke is a risk factor of space flight), a hormone for osteoporosis prevention (decalcification of bone), a toll-like receptor 5 agonists (radiation protection), and G-CSF (for neutropenia).
Once you leave Earth, there is no ionosphere for protection and the conditions are extreme. Solar radiation is very damaging, and there are also extremes of hot and cold. With Lynn, we discovered that spores are a very robust container for cell machinery and are resistant to the damage of space. Spores housed on the outside of the International Space Station for around three years were still able to regenerate and produce proteins. Nature never ceases to amaze me – and this is also why a lot of work has looked at extremophiles that have evolved in extreme environments, such as hypothermal vents, the Antarctic, or the bottom of the ocean. Now, we are combining spores with microfluidics as a way to handle and reconstitute them effectively. For the platform, you’ll be able to push a button to reconstitute the spores and then they will start protein production.
As well as ensuring the spores can survive in space, we also need to consider the technology that will be used to make the platform. It must be a self-contained unit, so that if something goes wrong it doesn’t cause a cascade failure that affects the spacecraft. It must also be robust, and capable of self-monitoring and correcting errors. The people that are using it aren’t going to be pharmacists or biochemists, after all.
And then we need to consider the purity of the protein therapeutic that is produced. When it comes to consistent quality and purity for biopharmaceuticals, even big pharma companies can struggle. Something that is in our favor though is that we are only making relatively small amounts of material, which makes purification much easier. When purifying a large batch of product, at times it can be like looking for a particular needle in a pile of different but ever-so-similarly looking needles. We are still working on purification methods but, ultimately, we hope to incorporate an automated quality control function into the system. We are very interested in antibody fragments and nanobodies because they can be produced using the cell-free system. We would express the protein we want, and also the antibody for that protein, with the latter being used to separate out the protein. Both protein and nanobody would have to be folded correctly and functional for the separation to work, and in this way only correctly folded and functional biologics would be outputted from our system. It is early stage, but we are making progress.
A bold new field
Our astropharmacy field is growing and every corner we turn opens up many new directions. It’s a very exciting field to be working in. We currently have seven PhD students working on a variety of projects, including:
- On-site, on-demand manufacture. Linked to the EPSRC project mentioned earlier, this project is studying various extremophiles as potential sources of environmental-tolerant cell-free protein synthesis.
- Drug delivery from halophiles. Exploring the particular and unique properties of halophiles, and whether we can reverse engineer novel drug delivery vectors based on their membranes.
- Cold plasma food processing. The nutritional requirements and ability to absorb nutrients of an astronaut changes in time. We are exploring the use of processing technology as methodology to modify the physical and nutritional content of food for personalized care.
- Bacterial/immune system crosstalk. It is known that both bacteria and the cells of the immune system change in microgravity. We are studying any interlink between the behavior of these two systems to research potential new methods to control infection and moderate disease.
- Insulin receptor dynamics and restorative treatment. Insulin receptors in muscle shutdown in spaceflight after a month. The same happens to immobile patients after a few days. This project is researching the biochemical processes that regulate receptor activity, and looking at potential pharmaceutical therapies and engineering solutions prevent or restore activity.
- CubeSAT design. Ultimately, research into how to make medicines and treat people in space needs to be done in the environment of spaceflight. Here, we are developing a flexible platform for astropharmacy experiments, based on a CubeSAT design. We hope to fly the first Astropharmacy CubeSat in February 2021.
The enthusiasm of the students is inspiring – and by focusing on the extreme problem of supporting life on Mars, I believe that we will, as a consequence, come up with many new solutions and approaches that can also benefit patients on Earth. In my view, astropharmacy is a new way to teach people to solve problems. It’s not about solving the particular problem, but translating problems to new environments. Because when you look at a problem from a new angle or in a new environment, you can often find new insight.
Meeting of Minds
With Phil Williams
Part of my research interest is understanding protein structure and function – one protein – titin – is found in muscle. We studied the mechanical properties of a part of this protein, I27, by pulling on the N and C termini, recording the force needed to unfold it. We also studied mutants of the same protein, probing how changes to individual amino acids affected the fold of the protein and thus its mechanical properties.
I then had the idea of seeing if we could make the same I27 mutants in actual muscle – to look for correlations between the mechanical strength of muscle and the mechanical properties of the protein – linking genotype and phenotype. My colleague, Nate Szewczyk (a professor of space biology at the university), was using nematodes (roundworms) as models for human muscle and flying them onto the SpaceLab and International Space Station to study how muscles atrophy and degrade in zero gravity. I asked for his help and it turned out we couldn’t at the time make the same single residue mutations inside the worm. But it did spark our collaboration – and my interest in space bioscience.
Our work on the mechanical properties of proteins developed into studies of how these properties evolved on Earth. Many proteins need to withstand force to function, and many protein systems are similar irrespective of the size of the animal. The process of blood clotting, for example, which is regulated by force, is the same in the giraffe as it is in the mouse, yet the mechanics of the blood flow are different. How have these individual proteins evolved their particular mechanical properties? We then moved on to in vitro evolution, where proteins are evolved in a test tube and selected for a particular property. Nobel Laureate Jack Szostak had evolved – from a random sequence of DNA, in a test-tube using cell-free synthesis – a protein with a particular function (binding ATP), which turned out to have a structure unlike any other protein on Earth.
I helped a postgrad student, Gareth Shannon, take a placement at NASA Ames to continue to study this remarkable protein and see if, via computer modeling, we could mutate the protein without losing function. If we could, it would suggest a whole universe of unique functional proteins out there that we could exploit. This points to both the origins of life and to immensely powerful tools for the future.
Gareth secured a post-doctoral position at NASA. At a similar time, I was discussing with colleagues the concept of Pharmacy 2.0. What will the profession of pharmacy look like in 50 years’ time? I mused on life on Mars and astropharmacy. How will Martian explorers receive medical care? I have been developing ideas ever since, talking to colleagues and collaborators (who often initially think I am mad!) along the way.
In June 2018, I visited Gareth at NASA Ames to give a talk (on work that Gareth had been involved in around protein evolution) and I met Lynn. We chatted for a good while, and I mentioned my thoughts on the challenges of pharmaceutical care on Mars. And that’s when we started working together.
Read more about making medicines in space: