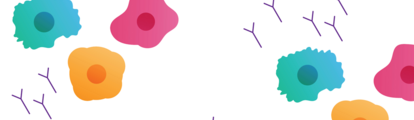
Fighting Viruses with Enhanced ADCC
With evidence mounting for the importance of antibody-dependent cellular cytotoxicity in controlling viral infections, mAbs engineered to enhance the effect are primed for success
Andrew J Racher |
sponsored by Lonza
Antibodies form an important feature of the host adaptive immune response to viral pathogens. Passive administration of antibodies obtained from convalescent patient plasma is a well-established concept (1), for example, as part of the treatment regimen following exposure to rabies virus. Building upon this concept, a number of recombinant mAbs have been developed over the last 25 years for treating viral infections (2). At the time of writing, there are six antibody therapeutics approved for the treatment of viral infections. Two of these antiviral antibody therapies are cocktails of at least two mAbs. As of early November 2020, there are sixteen mAbs in clinical trials for the treatment of COVID-19 that target the SARS-CoV-2 S protein (3). Three of these mAbs are Fc-modified to reduce the likelihood of antibody-dependent enhancement of virus infection, to extend half-life, or to enhance binding to immune-activating receptors.
Researchers are always looking for new ways to improve effectiveness of antibody therapy – often by engineering cells to enhance certain immune mechanisms. One such promising mechanism is antibody-dependent cellular cytotoxicity (ADCC).
Following the initial virus challenge, there are a number of processes – such as neutralization and agglutination – by which antibodies directly prevent viral infection. Binding of a virus-antibody complex to an Fc receptor on a phagocyte can trigger phagocytosis, resulting in destruction of the virus (see Figure 1). Binding to the Fc receptors on immune effector cells, such as monocytes, neutrophils, eosinophils and NK cells, trigger the release of cytotoxic factors (for example, antiviral interferons), creating a microenvironment that is hostile to virus replication. Various research groups (4,5,6,7,8) have demonstrated that broadly-specific neutralizing mAbs targeting highly conserved regions of haemagglutinin (a glycoprotein found on the surface of influenza viruses and integral to their infectivity) require Fc-mediated effector functions, especially ADCC, to protect against influenza virus challenge in vivo. Therefore, enhancing the potency of ADCC could be an effective way of combating influenza virus infection.
ADCC may be important in controlling other viral infections too. For example, researchers have successfully treated HIV-1 with broadly-specific mAbs in animal models (9,10,11). They attributed this success to both the neutralization function of the anti-HIV-1 mAbs, as well as their ability to activate ADCC – which removes infected cells. ADCC is also considered a potentially important mechanism for protective immunity in herpes simplex virus infections (12). It has also been shown that serum antibodies against hepatitis C virus E2 protein can mediate ADCC (13,14). Taken as a whole, there is growing evidence that ADCC is a significant component of the adaptive immune response to virus infection, highlighting the importance of strategies that induce and enhance ADCC in vaccine development.
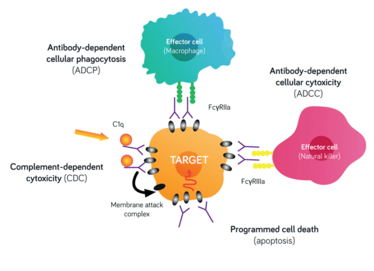
Figure 1. Antibody modes of action. Cell lysis through activation of complement dependent cytotoxicity (CDC); interaction with Fc receptors on effector cells to engage antibody dependent cellular cytotoxicity (ADCC); signaling for ingestion of a pathogen or target cell by a phagocyte.
Enhancing ADCC
How might researchers go about enhancing ADCC? The principal receptor for NK cell-mediated ADCC is the low affinity Fc gamma receptor, FcγRIIIa (CD16a). FcγRs bind to a region in the hinge and proximal CH2 region within the Fc fragment of antibodies, with IgG1 and IgG3 interacting most efficiently. This interaction is critically dependent on the glycan at the N297 site in the CH2 region (15). Removal of the entire N-glycan structure reduces the binding of Fc to FcγRs, and reduces or eliminates the associated effector functions. Conversely, eliminating only the fucose residue from the core glycan structure creates an afucosyl mAb and strengthens the binding of a mAb to FcγRIIIa receptors, enhancing ADCC (16,17,18). The strength of Fc-FcγR interactions can also be modulated by mutating the Fc region (19).
This mechanism also has support from Desheng Kong and colleagues, who showed that an anti-HIV-1 afucosyl-bispecific antibody had a greater ability to kill HIV-1 infected cells through ADCC than the fucosylated form (20). Another study found that enhanced ADCC was a potential mechanism for protection against Ebola virus infection, with afucosylated mAbs having up-to an approximately 11-fold lower ED50 than fucosylated mAbs (21).
The growing evidence for the importance of ADCC in controlling viral infections suggests that mAbs engineered to enhance ADCC (for example, through production in CHO FUT8 knockout variants of the host cell line (such as Lonza’s POTELLIGENT® CHOK1SV®) will become an important element of successfully treating viral infections. Recent studies (22,23) point towards the potential importance of strategies that target effector function pathways. Schäfer and colleagues (22) report that intact Fc effector function is required for protection from SARS-CoV-2 infection. Chakraborty and colleagues (23) report lower Fc fucosylation in antibodies to the receptor binding domain of the SARS-CoV-2 spike protein in adults with PCR-diagnosed COVID-19 compared to SARS-CoV-2-seropositive children and relative to adults with symptomatic influenza virus infections. This suggests strategies that target FcγRIIIa pathways may have therapeutic benefits. The enhanced ADCC activity of reduced fucose antibodies could contribute to viral clearance through killing and removal of infected cells. As overactive ADCC function may exacerbate symptoms in some cases, it will be important to carefully dose mAbs with high levels of effector function. Recombinant low fucose or afucosyl mAbs produced using CHO cells would form an important element of a strategy to produce mAbs with high-level ADCC effector function.
The adaptive immune response to virus infection is complex, involving the production of a broad repertoire of antibodies that interact with a range of Fc- and other receptors. As such, potent Fc-engineered or glycan-engineered mAbs with enhanced ADCC activity may form part of an antibody cocktail that mimics the nuanced in vivo response to virus infection – and a number of mAb-therapies for treating virus infection are already cocktails of at least two mAbs. Such antibody cocktails simultaneously target non-overlapping epitopes minimizing the likelihood that the virus becomes resistant to treatment.
The field has come a long way since the approval of the first therapeutic mAb in the 1980s. Today, we may be witnessing, as Alicia Chenoweth and colleagues (24) have alluded to, the “next-gen” of antibody therapies, with researchers taking new findings from immunology and using them to tweak and improve existing treatments. These next-gen biologics may unlock the full potential of antibody immune therapies – bringing new and exciting treatments to patients with difficult- or impossible-to-treat diseases.
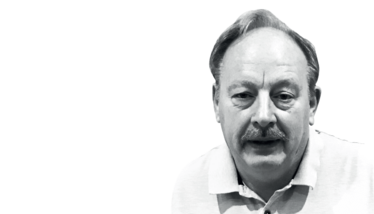
Dr Racher is a Director within R&D at Lonza Pharma, Biotech & Nutrition. Twenty-four of his 32 years working in the field of therapeutic protein production have been at LPBN. He has held technical and managerial roles in both Process Development and in R&D leading both technology and commercial development projects.
- A Hey, “History and Practice: Antibodies in Infectious Diseases,” Microbiology spectrum, 3, Aid-0026-2014 (2015).
- G Salazar et al., “Antibody therapies for the prevention and treatment of viral infections,” NPJ Vaccines, 2, 19 (2017).
- L DeFrancesco, “COVID-19 antibodies on trial,” Nat Biotechnol, (2020) doi.org/10.1038/s41587-020-0732-8.
- DJ DiLillo et al., “Broadly neutralizing anti-influenza antibodies require Fc receptor engagement for in vivo protection,” J Clin Invest, 126, 605-610 (2016).
- DJ DiLillo et al., “Broadly neutralizing hemagglutinin stalk-specific antibodies require FcγR interactions for protection against influenza virus in vivo,” Nat Med, 20, 143-151 (2014).
- W He et al., “Epitope specificity plays a critical role in regulating antibody-dependent cell-mediated cytotoxicity against influenza A virus,” Proc Natl Acad Sci USA, 113, 11931-11936 (2016).
- PE Leon et al, “Optimal activation of Fc-mediated effector functions by influenza virus hemagglutinin antibodies requires two points of contact,” Proc Natl Acad Sci USA, 113, E5944-e5951 (2016).
- DC Ekiert et al., “Antibody Recognition of a Highly Conserved Influenza Virus Epitope,” Science, 324, 246-251 (2009).
- NM Abuharfeil, MM Yaseen and FM Alsheyab, “Harnessing Antibody-Dependent Cellular Cytotoxicity To Control HIV-1 Infection,” ACS Infect Dis, 5, 158-176 (2019).
- J Carrillo, B Clotet and J Blanco, “Antibodies and Antibody Derivatives: New Partners in HIV Eradication Strategies,” Front Immunol, 9, 2429 (2018).
- WS Lee and SJ Kent, “Anti-HIV-1 antibody-dependent cellular cytotoxicity: is there more to antibodies than neutralization?” Current opinion in HIV and AIDS, 13, 160-166 (2018).
- S Görander et al., “Anti-Glycoprotein G Antibodies of Herpes Simplex Virus 2 Contribute to Complete Protection after Vaccination in Mice and Induce Antibody-Dependent Cellular Cytotoxicity and Complement-Mediated Cytolysis,” Viruses, 6, 4358-4372 (2014).
- Nattermann, J. et al., “Serum antibodies against the hepatitis C virus E2 protein mediate antibody-dependent cellular cytotoxicity (ADCC),” J Hepatol, 42, 499-504 (2005).
- L Long, and T Shen, “Natural killer cells in HCV infection,” J Clin Cell Immunol, 8, 1000530 (2017).
- H Liu et al., “Impact of IgG Fc-Oligosaccharides on Recombinant Monoclonal Antibody Structure, Stability, Safety, and Efficacy,” Biotechnol Prog, 33, 1173-1181 (2017).
- RL Shields et al., “Lack of fucose on human IgG1 N-linked oligosaccharide improves binding to human Fcγ RIII and antibody-dependent cellular toxicity,” J Biol Chem, 277, 26733-26740 (2002).
- Y Sakae et al., “Conformational effects of N-glycan core fucosylation of immunoglobulin G Fc region on its interaction with Fcγ receptor IIIa,” Scientific Reports, 7, 13780 (2017).
- T Shinkawa et al., “The absence of fucose but not the presence of galactose or bisecting N-acetylglucosamine of human IgG1 complex-type oligosaccharides shows the critical role of enhancing antibody-dependent cellular cytotoxicity,” J Biol Chem 278, 3466-3473 (2003).
- X Wang, M Mathieu and RJ Brezski, “IgG Fc engineering to modulate antibody effector functinons,” Protein & Cell, 9, 63-73(2018).
- D Kong et al., “A defucosylated bispecific multivalent molecule exhibits broad HIV-1-neutralizing activity and enhanced antibody-dependent cellular cytotoxicity against reactivated HIV-1 latently infected cells,” AIDS (London, England), 32, 1749-1761 (2018).
- L Zeitlin et al., “Enhanced potency of a fucose-free monoclonal antibody being developed as an Ebola virus immunoprotectant,” Proc. Natl. Acad. Sci. USA, 108, 20690-20694 (2011).
- A Schäfer et al., “Antibody potency, effector function and combinations in protection from SARS-CoV-2 infection in vivo,” bioRxiv (2020).
- S Chakraborty et al., “Symptomatic SARS-CoV-2 infections display specific IgG Fc structures,” medRxiv (2020).
- AM Chenoweth at al, “Harnessing the immune system via FcgammaR function in immune therapy: A pathway to next-gen mAbs,” Immunol Cell Biol, 98, 287-304 (2020).