From Brick Dust to Blockbuster
How can we best deliver solubility-challenged small molecules to the clinic?
David K. Lyon | | Longer Read

The small molecule drug development landscape is changing. The number of complex, highly potent compounds taken to clinical trials and market is increasing, largely driven by biopharma oncology and immunology portfolios (1). Furthermore, smaller companies drive much of the innovation – not only in taking molecules to the clinic, but also to commercialization – although they usually lack the “bricks and mortar” necessary to bring a compound to market alone (2). Additionally because of the breakthrough designations, many molecules successful in the clinic follow accelerated timelines. This can put additional pressure on defining the supply chain to meet product timing. Finally, many new products, whether they are first-in-class or fast-followers, face uncertain market demand once approved, which leads to difficulties in forecasting market uptake and peak market demand.
A simultaneous trend in small molecule development is the continuing increase in low-solubility compounds (3). Low-solubility compounds cannot be orally absorbed and therefore cannot have a therapeutic effect. There are many technologies drug developers can use to address molecule complexity and bioavailability challenges, but taking a trial-and-error approach to technology selection can be time-consuming and costly. A better approach is to analyze the optimal technology based on a compound’s physical, chemical, and biological properties. This method can help speed the delivery of more solutions to patients in need.
Working with the insoluble
Experts say as many as 70–90 percent of compounds in the pharmaceutical pipeline can be poorly water-soluble. The low solubility of these complex molecules can be driven by poor dissolution or can be truly solubility-limited due to either the lipophilicity of the molecule or high crystal forces that inhibit dissolution. These types of compounds are often referred to as “grease ball” or “brick dust” compounds.
To put the concept of low-solubility compounds into context, commercially marketed compounds such as itraconazole (~1–4 ng/mL soluble in water) and abiraterone acetate (~1 μg/mL) are approximately 10–1,000 times less soluble than the Venus de Milo – an ancient Greek statue made from marble that has not dissolved in around 2,000 years.
Numerous technologies are available to address solubility challenges, including particle size reduction to increase dissolution rate; complexation using cyclodextrins to increase effective solubility; lipid-based formulation (LBF) delivery; or amorphous solid dispersions (ASD) to increase both dissolution rate and solubility (5). Of these technologies, the most common in commercial use in the past decade are LBFs and ASDs (6).
Due to the vast number of potential technologies used to advance a compound, the pathway from preclinical drug substance to clinical-ready dosage form is complex and costly. This is especially true if it follows a trial-and-error approach to formulation and technology evaluation. Avoiding trial-and-error requires formulators to address a multifaceted problem – but this can be done by considering the molecule’s physical-chemical-biological properties (compound and property qualification), decision trees, high-throughput screening, in silico approaches, and the use of guidance maps (7, 8, 9, 10).
In principle, science-based methods can lead to rational formulation selection. However, methods based on fundamental knowledge of a compound’s physical-chemical-biological properties (compound and property qualification) or on extensive experience with a broad range of compounds (guidance maps) can lead to an optimal formulation faster. When using compound and property qualification, we consider an aggregate of compound properties (see Figure 1) to determine the appropriate formulation to progress.
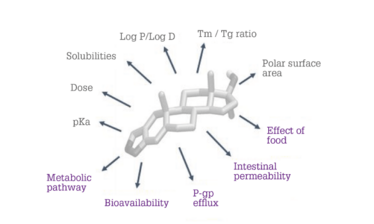
Figure 1. Physical-chemical-biological properties considered in a compound and property qualification scheme.
For example, if a compound has a high melting-point-to-glass-transition-temperature ratio, the compound is likely to crystallize rapidly. Therefore, an ASD is likely to be the technology of choice. However, if a compound is highly lipophilic or is transported via a P-glycoprotein efflux mechanism, then an LBF would be more appropriate.
The guidance map approach uses physical-chemical properties to map technologies onto a chemical space. The map in Figure 2 plots aqueous solubility as a function of lipophilicity and shows regions where specific technologies are expected to provide an optimal formulation. As noted above, highly lipophilic compounds, which often have high lipid solubility, tend to be more appropriate in an LBF formulation.
Two case studies illustrate the use of compound properties and guidance maps.
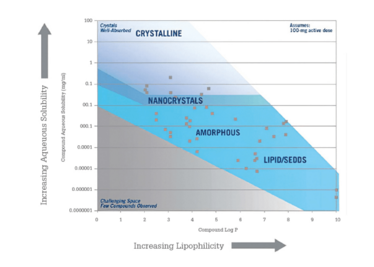
Figure 2. Guidance map showing technology applicability as a function of aqueous solubility and lipophilicity.
Case 1: LBF vs. ASD for a “grease ball” compound
Abiraterone acetate is a prostate cancer treatment marketed by Janssen Pharmaceuticals as Zytiga. The physical-chemical properties are listed in Table 1 and its position on a representative guidance map is shown in Figure 3.
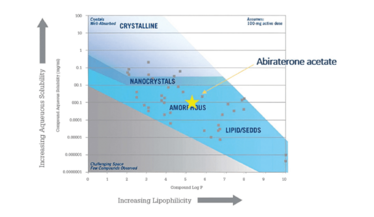
Figure 3. Abiraterone acetate plotted on a solubility-lipophilicity guidance map.
In Figure 3, it appears that abiraterone acetate would be preferentially formulated as an amorphous form. However, when the physical-chemical properties are examined, the compound’s lipophilicity, moderate melting point, low permeability, and positive food effect suggest that it may be a suitable candidate for LBFs.
To validate the technology selection criteria, abiraterone acetate was formulated using best practices as both an LBF and an ASD. These formulations were then evaluated using in vitro methods (see Figure 4) – a lipid digestion test for the LBF and a non-sink dissolution test for the ASD (11, 12).
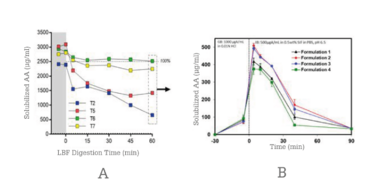
Figure 4. In vitro testing of abiraterone acetate LBP (A) and SDD (B) formulations.
An LBF was successfully prepared to sustain a supersaturated drug concentration for more than 60 minutes, whereas the ASD formulations became supersaturated and precipitated after about 45 minutes.
Based on these in vitro data, the best formulations from each technology class were implemented into a fasted beagle dog study. The results from the dog study are shown in Figure 5.
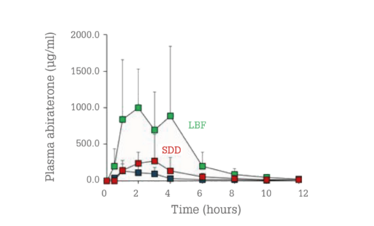
Figure 5. In vivo plasma concentrations for abiraterone acetate as a function of time in beagle dogs for LBF (green) and ASD (red) compared with the reference product (blue).
The LBF formulation’s performance was clearly superior to those of both the ASD and the reference product. The relative exposure increase was about 10-fold for the LBF and twofold for the ASD compared to the reference product. This emphasizes the need for careful evaluation of a compound’s physical-chemical properties when choosing the proper bioavailability-enhancing technology.
Case 2: The optimal spray ASD for a “brick dust” compound
In this case, research sought to determine the optimal formulation technology for itraconazole, marketed by Janssen Pharmaceuticals as Sporanox. Itraconazole appears to fall into a region that would favor an LBF (see Figure 6). However, the molecule’s low solubility in water, lipids, and solvents rules out a lipid solution and only allows for suspensions – which were significantly outperformed by the reference product in vivo in a rat study (13).
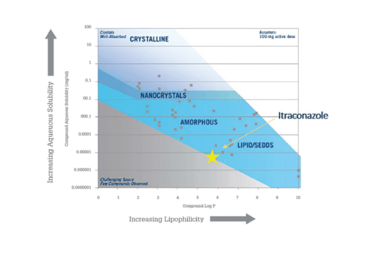
Figure 6. Guidance map of itraconazole as a function of solubility and lipophilicity.
Because the reference product was already formulated as a hydroxypropyl methylcellulose (HPMC) dispersion, the question became, “Could an improved amorphous dispersion be designed?” ASD formulations were prepared and evaluated using a material-sparing membrane permeability in vitro test (14). The itraconazole concentrations in the receiver phase of the membrane permeation test are shown in Figure 7.
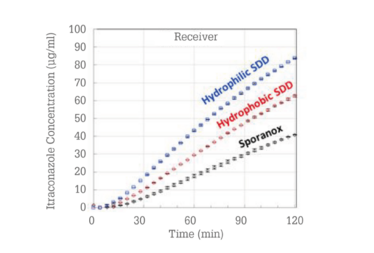
Figure 7. Membrane permeation results for three itraconazole ASDs.
Based on these tests, a hydrophobic and a hydrophilic ASD were taken into an in vivo rat study (see Figure 8). The hydrophobic and hydrophilic SDDs outperform the reference product by approximately 50 and 125 percent, respectively (15).
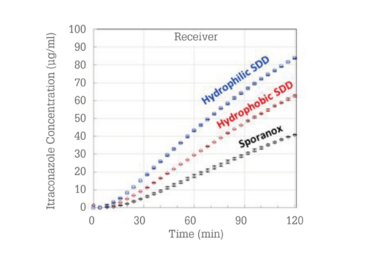
These results also demonstrate that in vitro technology selection methodologies can predict superior-performing bioavailability enhancement technology based on a compound’s properties and technology selection guidance maps.
These results also demonstrate that in vitro technology selection methodologies can predict superior-performing bioavailability enhancement technology based on a compound’s properties and technology selection guidance maps.
Choosing your tech
Oral bioavailability-challenged molecules are still present in large numbers in pharma and biotech pipelines. Multiple technologies can improve compound oral bioavailability, with LBFs and ASDs often most appropriate for grease ball compounds (such as abiraterone acetate) and ASDs for brick dust compounds (such as itraconazole). But, for each compound, we need rational technology selection to optimize performance, smooth development, and avoid overuse of precious resources. Validating a technology selection process also requires in vivo data – and there is currently no single in vitro method that can adequately compare all the different technologies.
- G Miglierini, “Emerging trends for the pharmaceutical market”, (2019). Available at: https://bit.ly/2Thp8EL
- 2. Lonza Internal Market Analysis
- 3. BJ Boyd, “Successful oral delivery of poorly water-soluble drugs both depends on the intraluminal behavior of drugs and of appropriate advanced drug delivery systems,” Eur J Pharm Sci., 137 (2019).
- 4. S Kalepu and V Nekkanti, “Insoluble drug delivery strategies: review of recent advances and business prospects”, Acta Pharma Sin B,5, 442, (2015).
- 5. HD Williams et al., “Strategies to Address Low Drug Solubility in Discovery and Development”, Pharmacol Rev, 65, 315, (2013).
- 6. S Ali and K Kolter, “Challenges and Opportunities in Oral Formulation Development”, Pharm Rev, 15, 1, (2012)
- 7. GA Fridgeirsdottir et al., “Support Tools in Formulation Development for Poorly Soluble Drugs”, J. Pharm Sci, 105, 2260, (2016).
- 8. W-G Dai et. al., “Advanced screening assays to rapidly identify solubility-enhancing formulations: high-throughput, miniaturization and automation,” Adv. Drug Delivery Rev. 2008, 60 (6) 657-672 (2008).
- 9. CA Bertrom et al., “Early pharmaceutical profiling to predict oral drug absorption: current status and unmet needs”, Eur J Pharm Sci, 57, 173, (2014).
- 10. DT Friesen et al., “Hydroxypropyl Methylcellulose Acetate Succinate-Based Spray-Dried Dispersions: An Overview”, Mol Pharm, 5, 1003, (2008).
- 11. B Wu, J Li, Y Wang, “Evaluation of the Microcentrifuge Dissolution Method as a Tool for Spray-Dried Dispersion,” The AAPS Journal, 18 (346-353 (2016).
- 12. HD Williams et al., “Toward the establishment of standardized in vitro tests for lipid-based formulations, part 1: method parameterization and comparison of in vitro digestion profiles across a range of representative formulations”, J Pharm Sci, 101,3360, (2012).
- 13. Y Sahbaz et al., “Transformation of poorly water-soluble drugs into lipophilic ionic liquids enhances oral drug exposure from lipid based formulations”, Molecular Pharmaceutics, 12, 1980, (2015).
- 14. AM Stewart et al., “Development of a Biorelevant, Material-Sparing Membrane Flux Test for Rapid Screening of Bioavailability-Enhancing Drug Product Formulations”, Mol Pharm, 14, 2032, (2017).
- 15. AM Stewart et al., “Impact of Drug-Rich Colloids of Itraconazole and HPMCAS on Membrane Flux in Vitro and Oral Bioavailability in Rats, Mol Pharm, 14, 2437, (2017).