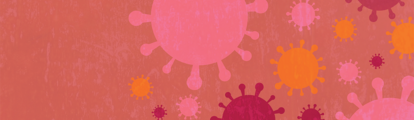
Protect the Virus!
Viral vectors can enable innovative medical treatments, but only if we address the unstable elephant in the room with a comprehensive formulation development plan
Gideon Kersten, Daniel Weinbuch, Tim Menzen, Andrea Hawe | | Opinion

Gideon Kersten, Scientific Reviewer and Advisor for Coriolis Pharma and an Extraordinary Professor of Vaccine Development, University of Leiden

Daniel Weinbuch, Business Development Manager for Coriolis Pharma
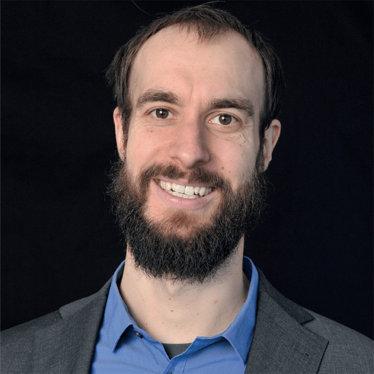
Tim Menzen, Chief Technology Officer at Coriolis Pharma
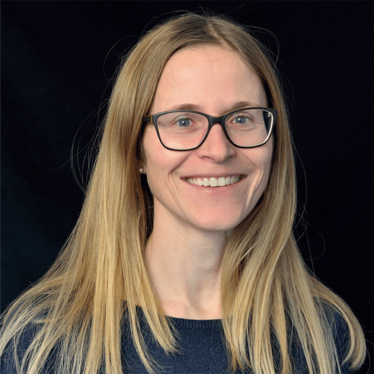
Andrea Hawe, Chief Scientific Officer at Coriolis Pharma
Viable viruses are an important group of biopharmaceuticals – and likely the oldest. As early as the 16th century, the practice of variolation was applied in India to combat smallpox. Dried pus from pustules from smallpox patients was administered to the skin of healthy people, unknowingly using viruses as medicines. This primitive and dangerous form of vaccination with a crude preparation of live smallpox virus provided some protection against smallpox. At the turn of the 18th century, Edward Jenner described the potential of less dangerous cowpox material to protect against smallpox. A century later, Louis Pasteur pioneered attenuation of infectious agents, including viruses, to use them as vaccines. All this is remarkable, when you consider that viruses were not discovered until the 1930s, when the development of filters allowed us to isolate viruses and the invention of the electron microscope finally allowed us to visualize them.
Since the 1990s, the use of viruses as gene delivery vehicles has taken off; hundreds of clinical trials have been performed and several dozen gene therapy products are now approved – and many of them use viral vectors. These can be applied directly to the patient (in vivo gene therapy) or used to transfect cells outside the body of the patient (ex vivo) before being returned to the patient.
A third therapeutic application of viruses is tumor targeting. Oncolytic viruses are made native or modified to specifically infect and destroy tumor cells and/or to stimulate anti-tumor immune responses. Several such products have been marketed since the first therapy, Rigvir, was approved in 2004 in Latvia, and numerous clinical trials are ongoing.
The origins of virus instability
As with all complex biological systems, viruses are intrinsically unstable. The loss of viability observed for viruses can be caused by:
1. Protein deterioration that prevents the binding of the virus to the receptor and/or destabilizes the protein capsid in the case of non-enveloped viruses. For instance, after a short treatment of poliovirus or vaccine at 56oC, the structure of the capsid changes, resulting in virus-like particles that can no longer bind to the receptor.
2. Damage to genetic material (DNA or RNA). RNA is particularly prone to hydrolysis in the presence of water, and at elevated temperatures it may lose the critical secondary structure of its regulatory elements.
3. Damage to the lipid membrane in enveloped viruses. For instance, the stability of retroviruses depends on the composition of the viral membrane, and therefore on the type of production cell line used (1).
4. A combination of all the above. For example, the viral genome is protected not only by a proteinaceous capsid and/or a lipid envelope, but may also contribute to the structural integrity of the virus (2). Therefore, the size of the genome of a viral vector should not be very different from the native genome. Also, the manner in which the DNA is packaged – dense or less dense – has an impact on the viral vector’s stability. Higher ‘DNA pressure’ may result in less stable virus and, as a result, lower infectivity (3).
The relative contribution of these factors to virus destabilization during processing (for example, freezing or drying) and storage is not well understood (4). But it is probably safe to say that viruses can and will deteriorate in any number of ways.
The development of stable virus formulations intended for human application first requires a clear target product profile (TPP) that defines, among other things, the route of administration, dosing, and primary packaging. Second, scientists must establish a set of stability-indicating and phase-appropriate analytical methods to identify and monitor critical degradation pathways and prove activity of the virus. Third, the laboratory infrastructure and analytical methods need to fulfill certain biosafety regulations for virus-based medicinal products; often a biosafety level (BSL) 2 is required. Last, but certainly not least, scientific expertise and prior knowledge in developing virus formulations and setting up analytical methods is extremely beneficial.
Liquid formulations – keep it cold!
The poor stability of viruses is the reason why the majority of currently licensed virus-based products are stored as frozen liquids at -20 °C or even lower temperatures. A few – Zolgensma is one example – can be stored at 2–8 °C for around 14 days. Oral polio vaccine is reasonably stable at 2–8 °C, but for storage periods exceeding 6 months, -20 oC or lower is advised.
As freezing may cause dramatic changes in ionic strength, osmolarity, and pH, the sensitivity of viruses to these effects should be investigated during formulation development, making it possible to select suitable conditions with respect to pH, buffers, and excipients. In addition, the effects of final storage temperature, freezing speed, thawing procedure, and so on should be determined experimentally. Knowing the physical state of a frozen solution as a function of temperature is important. Phase separation and other inhomogeneities in the matrix may occur during freezing. At moderately low temperatures such as -20 oC, solutions may not be completely frozen, leaving room for molecular mobility and chemical deterioration. Crystallization events and freeze concentration of excipients during freezing can damage the virus. Differential scanning calorimetry (DSC) reveals some of these effects and can help to select optimal freezing and storage conditions. In general, fast freezing rates are beneficial because they promote the formation of amorphous glasses instead of crystallized solids. In the case of enveloped viruses, fast freezing may avoid formation of lipid membrane damaging ice crystals (4).
Your selection of buffer and cryoprotectant is particularly important. Phosphate-based buffers may induce very considerable pH shifts of several pH units during freezing because of separate crystallization of the buffer salts. The main cryoprotectant groups are sugars, sugar alcohols, and alcohols.
The development of effective formulations often has a highly empirical nature. It is all but impossible to reliably predict the optimal compositions or concentrations of stabilizing excipients. The number of variables is large (consider type and concentration of excipients, freezing rate, thawing rate, combined effects of excipients, and so on), making it hard to perform extensive screenings that cover all aspects.
Therefore, a systematic and stepwise approach is highly recommended to generate a scientific understanding and to de-risk the development: starting with a pH/buffer screening, followed by an excipient screen with a selected cryoprotectants and other stabilizers, followed by an optimization phase in which, for instance, different excipient concentrations are tested. One complication with frozen liquid formulations is that accelerated stability studies are intrinsically impossible. Time-consuming real-time stability studies (apart from repeated freeze-thawing) are therefore the only way to assess stability. Despite these challenges, having experience in formulation of different viruses is beneficial to the setup of a scientifically sound and knowhow-driven formulation development approach. Moreover, multi-disciplinary teams of formulation scientists, analytical specialists, and virologists can increase success rates considerably.
The lyophilized “solution”
Supply chains with sub-zero temperatures are not always feasible. If frozen liquid formulations will not work, lyophilization can be used to stabilize the virus – indeed, this process is used for most live attenuated viral vaccines, including vaccines against measles, yellow fever, and rabies. However, it is important to design a formulation that protects the virus against potentially harmful events during freezing and drying. Typically, excipients with cryoprotecting and lyoprotecting activity must be present. Efficient lyoprotectants, such as sucrose and trehalose, are good water substitutes, which maintains conformation of viral proteins in the dried state. Lyoprotectants also contribute to a high glass transition temperature (Tg) of the lyophilized material, which is advantageous for storage. When the product is stored at temperatures above the Tg, the glassy state of the freeze-dried matrix becomes more rubbery, causing increased molecular mobility. In addition, recrystallization of amorphous excipients may occur, which may damage virus particles. Note that even small amounts of residual water will reduce the Tg significantly, rendering the product less stable. In short, it is important to keep water content low and to optimize the lyophilization process accordingly. In fact, the lyophilization process (the unit operation) must be developed and aligned with the formulation development process (the composition). Freezing rate, drying temperatures and pressures, and the application of controlled nucleation to reduce inter-vial differences in freezing rate all need to be considered.
Analyzing virus quality and stability
To assess the effect of formulation and storage conditions on product quality and virus stability, you’ll need appropriate analytical assays, which can be categorized as functional, semi-functional, and non-functional (see Table 1). Functional assays measure the potency of the virus, such as its ability to transfect cells or the immunogenicity of a live viral vaccine in experimental animals. These methods are usually time consuming, expensive, and not sufficiently accurate, which means they are less suitable for formulation screening purposes.
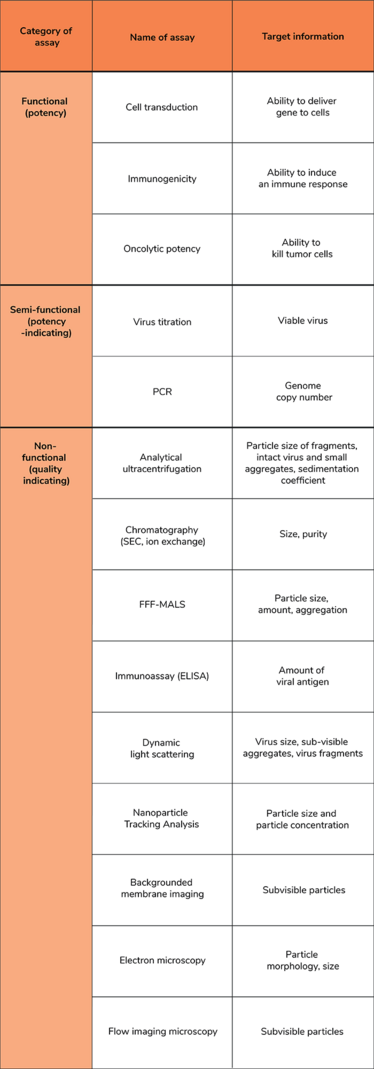
Table 1. Examples of analytical techniques for characterization of viruses (5). ELISA: enzyme-linked immunosorbent assay; FFF-MALS: Field-flow fractionation with multi-angle light scattering detection; PCR: polymerase chain reaction; SEC: size exclusion chromatography.
Alternative stability indicating assays for viruses are available. Infectivity assays are particularly important because they are semi-functional but less labor-intensive than functional assays. In general, the ability of viruses to infect cells is determined by measuring cytopathic effects (CPE) in host cells incubated with dilution series of the virus. Depending on the virus and the host cells, CPE can range from barely affected host cells to complete cell lysis. Readouts will differ depending on the type of CPE and may include direct microscopic visual assessment of the cells, immune fluorescence in a FACS, or fluorescent focus assays.
Infectivity assays, although very relevant, are still time consuming and lacking in accuracy. Instead, quantification of gene copy numbers by PCR is often used. This method does not measure viable viruses, but the number of viral genome copies. This may or may not correlate with infectivity.
A third group of assays are non-functional characterization methods. Despite the somewhat unappealing name, these assays can provide detailed information about the structural integrity of viral particles. Assays belonging to this category determine physico-chemical properties, such as particle size measurements by light scattering techniques, size exclusion chromatography, analytical ultracentrifugation, and AF4 (5). If necessary, viral components, such as proteins and nucleic acids, can be analyzed by electrophoresis, HPLC, and spectroscopic methods. The advantage of many of these techniques is that they are high throughput and generally more accurate and sensitive compared with the functional methods. The onset of viral aggregation or loss of virus – for example, due to adsorption – during a stability study is in some cases detected earlier than a statistically significant loss of virus titer. In this way, a more accurate ranking of formulations is possible, which in turn allows for rational selection of the best formulations.
Over the course of virus formulation development, a combination of functional, semi-functional, and non-functional assays is not only recommended – it is required.
We can do better
Formulation development of viral products is still highly empirical and, in our view, often not performed adequately. In that respect, one could argue that not much has changed since the 16th century’s variolations! The air-dried material from those times, high in potentially stabilizing impurities, may have been as stable (at least for a short period of time) and effective as some of today’s virus formulations.
In many cases, there is room for improvement – particularly when aiming for a high quality product with long-term stability. To achieve this goal, you should not rely on off-the-shelf formulations for the virus of interest and expect them to result in an acceptable stability profile. Viruses are highly sensitive, and – depending on the type of virus – different stability challenges may arise. To obtain a stable product, you must perform dedicated virus-specific formulation development from initial screenings to formulation optimization (including the proper set of analytical methods).
Scientific knowledge about factors influencing virus stability is growing – and so is our collective ability to overcome virus instabilities with science-driven formulation development. In the years ahead, we should be using expert know-how and applying a range of formulation approaches, including lyophilization, to obtain stable and phase-appropriate virus formulations.
Credit Figure: Servier Medical Art / smart.servier.com
- C Beer et al., “The temperature stability of mouse retroviruses depends on the cholesterol levels of viral lipid shell and cellular plasma membrane”, Virology 308, 137-146 (2003). DOI: 10.1016/s0042-6822(02)00087-9
- B Saha et al., “The adenovirus genome contributes to the structural stability of the virion”, Viruses 6, 3563-3583 (2014). DOI: 10.3390/v6093563
- DW Bauer et al., “Influence of internal DNA pressure on stability and infectivity of phage λ”, J Mol Biol 427, 3189-3200 (2015). DOI: 10.1016/j.jmb.2015.07.023
- IJJ Hansen et al., “Freeze-drying of live virus vaccines: a review”, Vaccine 33, 5507-5519 (2015). DOI: 10.1016/j.vaccine.2015.08.085
- A Roesch et al., “Particles in biopharmaceutical formulations, part 2: an update on analytical techniques and applications for therapeutic proteins, viruses, vaccines and cells”, J Pharm Sci 111, 933-950 (2022). DOI: 10.1016/j.xphs.2021.12.011
Gideon Kersten is a Scientific Reviewer and Advisor for Coriolis Pharma and an Extraordinary Professor of Vaccine Development, University of Leiden
Daniel Weinbuch is Business Development Manager for Coriolis Pharma
Tim Menzen is Chief Technology Officer at Coriolis Pharma
Andrea Hawe is Chief Scientific Officer at Coriolis Pharma