Seeing the Light
Optogenetics could lead to new ways of delivering drugs to the brain, central nervous system and spinal cord – and soluble circuits could have a role to play too
For the last 15 years, my research group at Northwestern University has been applying materials science concepts to drug delivery applications. Our aim is to unlock the potential of functional electronic devices, such as sensors, by integrating them with the human body. This approach should enable functionality far beyond anything found in conventional electronic implants, such as cardiac pacemakers, but requires very sophisticated technology and manufacturing techniques.
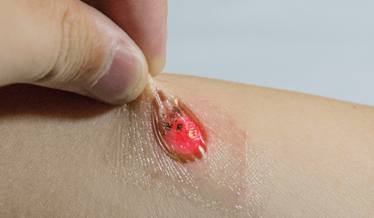
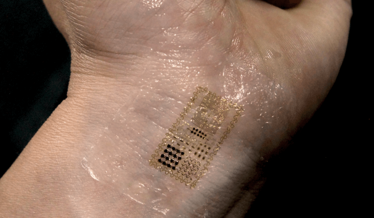
In particular, my group has a strong focus on neuroscience. Since about 2010, we – along with colleagues at Washington University Medical School, St Louis – have been working on a device that uses light-emitting diodes (the size of individual neurons) that activate brain cells with light. Optogenetics – using light to stimulate targeted pathways in the brain – allows neuroscientists to identify and map brain circuits in behaviors from depression, to addiction, to anxiety and more, which could in turn lead to new treatments.
One of my group’s focuses has been on improving the technology available for delivering light to the brain. When we started, the only option was a fiber optic telecommunication cable – a glass cylinder inserted into the brain so as to channel light to the neurons. This technique is invasive, damaging, and inconvenient as it physically tethers the subject to the light source. Our solution was to develop cellular-scale, battery-free, light-emitting diodes (LEDs) that can be embedded in the brain and wirelessly controlled. We mount the diodes on very thin, flexible plastic filaments that are much smaller and more biocompatible than fiber optic cables.
Make It So
How easy will it be to bring the new dosage form developed by Traverso and colleagues to market? Lyndra, Inc. (Watertown, MA, USA) was formed to commercialize the gastro-resident system. Here, we catch up with Ray Knox (Senior Vice-President of Manufacturing) and Ellie McGuire (Head of Business Development) to hear about the company's recent progress.
What stage are you at with this innovation?
RK: We are starting human trials this fall. We’ve done extensive testing of our dosage form in both porcine and canine models – well over 300 animals in total. We can’t release the animal data yet, but we anticipate using the information to support our first-in-human trial towards the end of this year. For our lead candidate, we will be pursuing a 505(B)(2) pathway.
What challenges has Lyndra faced?
EM: The main challenge faced by the inventors of this technology – Traverso, Langer, and Bellinger – was to achieve gastric residence and thereby permit ultra-sustained drug release. For a number of years, large companies have tried to solve the medication compliance issue by increased gastric residence. We’ve solved that problem in a new way, which was both our major challenge and our major achievement.
RK: That broad challenge was comprised of many smaller hurdles, like ensuring appropriate release kinetics over the duration of gastric residence. It required careful preformulation and formulation work to develop a blend of materials that will give us both the flexibility and tunability to achieve the desired target product profile for a range of compounds. It’s always challenging to control sustained drug release in a variable environment like the stomach, but we are making progress. As this dosage form is novel, its manufacturing is a major area of focus for us; however, we do not anticipate any major manufacturing obstacles going forward.
Have you discussed your approach with regulators?
RK: Yes, the regulators love to get discussions going at an early stage! Certainly these conversations are ongoing, and they have been very positive, but we are still early in the process. Nevertheless, I can say that these preliminary discussions have not identified anything that would be a significant challenge from a clinical trial or drug approval perspective.
What commercialization strategy do you anticipate?
EM: We have a dual-track business model. We are developing internal product candidates, and at the same time we are working with strategic external partners. In addition, we are collaborating with several institutions, and we hope these efforts will lead to globally accessible products suitable for resource-poor countries.
We are now extending our research to devices that can deliver drugs to targeted anatomical regions. Our recent paper demonstrates the advantages of combining our micro-scale LEDs with an ultra-miniaturized fluidics system fabricated from a flexible, biocompatible elastomer (1). This device allows us to deliver both drugs and light to optogenetically-controlled neurons, enabling simultaneous or sequential pharmacology and optogenetic control – in other words, we can study the neurological effects of new drugs at the point of delivery. This work was something of a technology milestone and is something the group is very proud of.
In the initial microfluidics embodiment, the drug reservoir is located in a helmet-like casing that fits on the head; from here, a thermal pump sends drug to the implanted microfluidic device. However, the thermal pumping mechanism is not ideal for temperature-sensitive APIs. We are now developing low temperature pumping mechanisms that are much more power-efficient and compact. This should allow integration of the pump and the drug reservoir in a single sub-dermal implant, enabling us to move away from the external helmet. We intend to deploy this triggered-release capability in the brain, spinal cord and peripheral nervous system.
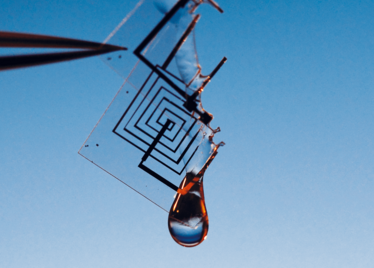
Soluble circuits
A consequence of the size of our operation (my group includes 25-30 post-docs, 15 graduate students, 30-50 undergraduates, and maybe 12 visiting scientists), is that at any one time we’re always pushing forward with multiple projects. Devices under development include skin-mounted appliances to continuously measure blood pressure, or to capture, transport, store and analyze sweat (to benefit exercise physiology). Another project involves a closed-loop feedback system such that a drug-containing device is autonomously triggered to release medication according to measurements it makes in the patient. The device sends data to a remote computer that carries out analytics and instructs the device to actuate the outlet and release the drug as appropriate. One near-term application for this device is bladder dysfunction.
One of our newest streams of research is “transient electronics” – biocompatible electronic systems that can dissolve in biofluids over a well-defined time period. It’s the same concept as a bioresorbable suture, but applied to a fully integrated electronics system, comprising a power supply, radio transmission capabilities, an electrical stimulation capability, and sensors. One example is our intracranial pressure monitor for tracking the recovery of patients who have suffered traumatic brain injury (2). The device is implanted in the intracranial space. Normally, you would need secondary surgery to remove the device once the patient has recovered, but our device is simply resorbed over time. This notion of water-soluble electronics is very exciting; it could have applications not just in the biomedical field, but also in the environmental arena; for example, in helping to deal with the discarded electronics component of toxic waste streams. We are bringing this field forward very rapidly now.
Finally, in addition to needle-based fluidic systems, which deliver drugs by injection, we are developing devices with release valves that can be triggered to open as required – the drug is then released from a reservoir by diffusion. Again, these systems are wirelessly controlled, implantable and resorbable. Once the drug reservoir is empty, it is naturally eliminated.
In summary, the field has great potential and has picked up tremendous momentum. Many other groups are now getting involved, to the point where the concept of “bio-electronic medicines” is almost mainstream. Super-miniaturized, biocompatible devices allow you to treat disease in a way that is complementary to pharmacotherapy – think of devices that can interface with peripheral nerves to manage pain, or be applied to wounds to accelerate healing.
John Rogers is Louis Simpson and Kimberley Querry Professor of Materials Science and Engineering, Biomedical Engineering, Mechanical Engineering, Electrical Engineering and Computer Science, Chemistry and Neurological Surgery; and Director of Center of Bio-integrated Electronics Northwestern
University, US.
- JW Jeong et al., “Wireless optofluidic systems for programmable in vivo pharmacology and optogenetics”, Cell, 162, 662-674 (2015). PMID: 26189679.
- SK Kang, et al., “Bioresorbable silicon electronic sensors for the brain”, Nature, 530, 71-76 (2016). PMID: 26779949.
John Rogers is Louis Simpson and Kimberley Querry Professor of Materials Science and Engineering, Biomedical Engineering, Mechanical Engineering, Electrical Engineering and Computer Science, Chemistry and Neurological Surgery; and Director of Center of Bio-integrated Electronics, Northwestern University, US.