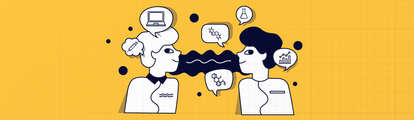
The Case for MMC
The use of multimodal chromatography is increasing in biopharma purification processes. Here’s why.
Heidi Jones | | 8 min read | Practical
Multimodal (or mixed-mode) chromatography (MMC) is increasingly being used in the biopharma industry for purification. The technique allows for a broader range of ligand interactions with the target molecule, resulting in enhanced selectivity and purification capabilities compared with traditional chromatography methods.
In resin format, MMC uses ligands on the stationary phase that exhibit two or more interaction modes, such as hydrophobic, electrostatic, hydrogen bonding, and metal affinity. This approach enables the manipulation of the mobile phase to maximize multimodal ligand interactions with the target biomolecule and remove impurities, such as host cell proteins and DNA.
From a process development and business perspective, MMC has the potential to improve overall purification process efficiency by combining at least two interaction modes in one step. Ultimately, this improvement in efficiency can translate to reduced costs while increasing yields.
The rise of multimodal
Multimodal purification is not new. Indeed, many traditional monomodal resins exhibit multimodal interactions that are caused by attributes of the base bead matrix used to secure the ligand of interest (1).
Calcium hydroxyapatite (CHT), for example, is an inherently multimodal resin that has been widely used since the 1950s for a variety of bioprocessing applications, including mAbs, vaccines, separation of single-stranded from double-stranded DNA, antibody-drug conjugate (ADC), and bi-specific downstream processing (1, 2, 3, 4). CHT is a chemically synthesized ceramic bead composed of both phosphate and calcium groups, capable of both metal affinity (Ca2+) and cation exchange (CEX) interactions due to its phosphate residues. Manipulating salt and/or phosphate concentrations of the mobile phase enables selective elution of impurities and target biomolecules.
The predecessor of today’s MMC resins were born from observations in the resolution differences of nucleic acid separation, which could not be explained by ligand chemistry alone. Differences were observed with different base matrices, despite using the same stationary phase ligand motifs; for instance, diethylaminoethyl cellulose (DEAE) bound to cellulose exhibited different resolution capabilities to DEAE bound to agarose. Other multimodal observations were also made, such as the effects on resolution due to free silanol groups on silica-based matrices in reverse-phase (RP) chromatography, retention affects caused by hydrophobic interactions in ion exchange (IEX) and affinity chromatography media, and the effects of electrostatic interactions on size exclusion chromatography (SEC).
In early nucleic acid separation using HPLC, anion exchange (AEX) resins were widely used, with alkyl amine-bound matrices enabling separation of nucleic acids (for example, plasmid DNA, viral DNA, or DNA restriction fragments) from biological samples based on interaction with negatively charged phosphodiesters. HPLC grade-silica-based bead technology was later developed and designed to withstand higher pressures and flow rates.
Secondary weaker matrix interactions were initially seen as detrimental in chromatographic separations. It wasn’t until the 1960s that researchers made the first true attempt to make an MMC resin for HPLC applications, designed to enhance nucleic acid separation by using AEX attributes and the presence of lysine and arginine side chains, alongside secondary effects caused by methyl groups (2).
Mixed-Mode Ligands
Hydrophobic, anionic ligand with hydrogen bonding – this ligand features hydrogen bonding, a quaternary amine, and a phenyl group.
Mixed-mode cationic ligand with hydrophobic binding – this ligand contains a secondary amine and is cationic over a wide pH range; therefore, it behaves as both a hydrophobic interaction resin and an anionic exchange media. At low ionic strength, it can bind acidic proteins as well as proteins with moderately high isoelectric points. However, hydrophobic interactions predominate, as binding capacity increases with temperature and salt.
Mixed-mode pH-controllable sorbents – this ligand contains a 4-mercaptoethylpyridine (MEP ligand. The pyridine ring is uncharged at neutral and basic pH. As the pH decreases, the pyridine nitrogen becomes positively charged, turning the resin into a mixed-mode media. MEP becomes a pH-controlled mixed-mode ligand, a property that has been termed “hydrophobic charge induction chromatography” (Burton and Harding, 1998).
Example of mixed-mode ligands. A is a hydrophobic, anionic ligand with hydrogen bonding. B is a mixed-mode pH-controllable sorbent. C is a mixed-mode cationic ligand with hydrophobic binding.
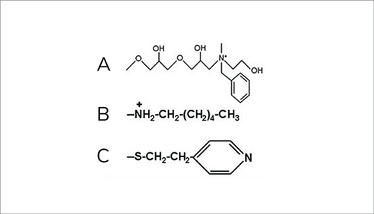
Figure 1. Examples of multimodal ligand chemistries (5)
Bioprocessing benefits
MMC has now extended beyond its early nucleic acid applications into large scale bioprocessing, with advances in predictive screening processes enabling more rapid analysis of MMC resins for biomolecule separation. High throughput techniques, such as design of experiment plate screening and, more recently, advanced mechanistic modelling, can be used to predict the optimal mobile phase conditions for a particular biomolecule separation (1, 6).
Because MMC allows for a broader spectrum of ligand interactions, it is particularly useful when purifying highly charged or polar molecules – or those exhibiting salt or pH sensitivity, which can make separation using reverse phase, ion exchange, or affinity liquid chromatography especially challenging. Additionally, the simultaneous manipulation of multimodal interactions can achieve increased biomolecule resolution, a valuable feature not only in bioprocessing, but also in HPLC applications for analytical scale bio-separations and impurity analysis (7).
MMC has several advantages over monomodal chromatography (1). Firstly, MMC offers multiple interaction modes, which provides greater versatility and high selectivity for a wide range of target molecules, such as bi-specific antibodies, acid- and salt-sensitive antibodies, and ADCs. Multimodal ligands may enable higher target binding capacity over traditional monomodal methods, leading to more efficient capture and purification.
MMC offers a ‘gentle’ solution for the purification of sensitive molecules, minimizing the denaturation and aggregation of target proteins during the process. The ability to purify multiple targets in a single step can reduce the number of purification stages, resulting in cost, time, and resource savings.
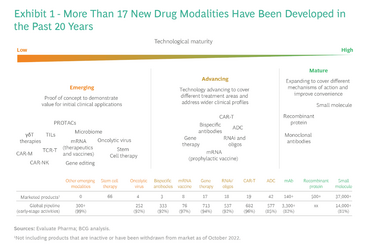
Figure 2. Benefits and risks of new drug modalities (8)
MMC: Why fix what’s not broken?
Despite its advantages over current monomodal methods, MMC faces several barriers that make entry into the biopharmaceutical manufacturing space difficult.
For example, current manufacturing processes for therapeutic mAbs that rely on sequential monomodal purification are well developed, validated, and firmly established. Why change something that already works? Introducing multimodal methods would require an effective case to convince upper management and investors that the newer, less established technology is a superior alternative to standard purification processes.
Another drawback is that the selectivity of MMC varies depending on the target molecule and its interaction with the ligands, which can make purification unpredictable. Some MMC resins may also have limited stability under certain conditions, affecting their performance and reusability – and potentially increasing operational costs. Depending on the chromatography system, MMC ligands can also be prone to leaching from the matrix, resulting in contamination of the purified product.
These are not insignificant issues; however, in a world where mAbs dominate the therapeutic landscape – and with continual boosts to upstream processing – MMC looks like an increasingly attractive solution. After all, with increased protein production comes increased levels of aggregation and HCP impurities, which can make high purity and high yield more difficult to achieve (1).
In addition, many new drug modalities are coming to fruition (see Figure 2), which creates an exciting space for researchers to design unique downstream processes. Indeed, there has been a surge not only of new MMC resins, but also new media technologies (for example, multimodal membrane and multimodal monolith technology; see sidebar) that aim to deliver greater selectivity at a faster rate than current resins.
Downstream bioprocessing typically involves an orthogonal purification approach; for example, a mAb platform process can comprise an affinity capture step followed by viral inactivation and additional chromatography steps to ensure high product purity is achieved along with sufficient reduction in process impurities (see Figure 3). MMC has the potential to reduce this downstream processing workflow by combining two or more steps into one, improving purification efficiency whilst simultaneously reducing processing time and cost.
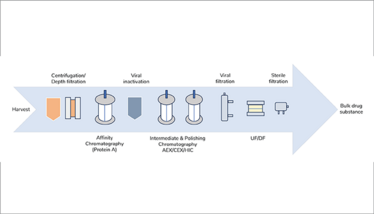
Figure 3. Overview of a typical mAb manufacturing process
It also seems likely that there will be a rise of MMC specifically designed to enhance mRNA and other oligo therapeutic separations. Although various resins have been developed over the last ten years or so years that preferentially bind to the poly A tail of in vitro transcribed mRNA to enable straight forward capture steps, secondary polishing steps are still required for additional purity enrichment.
The future of MMC is promising. As research and innovation continues, the theoretical basis and practical applications of MMC will become even better understood, broadening its use.
I believe that improvements in resin design will overcome issues related to ligand stability, leakage, and selectivity variation, improving the overall performance, reliability, and, therefore, adoption of MMC. Additionally, with a deeper understanding of multimodal interactions and ligand design, personalized purification strategies tailored to specific biomolecules or complex mixtures may become more common.
With advances in automation and robotics also enabling large-scale and high-throughput purification processes, MMC undoubtedly has huge potential as a powerful and versatile tool across biotech, pharma, and beyond.
Membrane and Monolith Technology
Recent advances in ligand and polymer technology have resulted in the development of single use membranes with higher dynamic binding capacities and improved selectivity, which can be used for the purification of a wide range of biomolecules in flowthrough mode, including viruses, mAbs, and endotoxins. Membranes have the advantage of significantly higher throughput over resins because of their flow properties.
A key obstacle in mAb bioprocessing is salt sensitivity. Load material must often be diluted to reduce conductivity before proceeding to the next step in a process. Multimodal membranes seek to bypass this processing bottleneck through the combination of IEX ligands (usually AEX) with hydrophobic, coulombic, and/or hydrogen bonding attributes to enhance salt tolerance while maintaining high binding capacity and selectivity (9).
Multimodal monolith technology has recently emerged that uses hydrogen bonding and AEX modalities (10). Specifically designed for the downstream processing of mRNA and virus separation, these technologies have the potential to meet demands for efficient high throughput processing and high selectivity.
However, both membrane and monolith technologies come with several drawbacks that may make wide scale adoption challenging. For monoliths, an inherent lack of homogeneity of pore size distribution throughout the column creates scaling issues, complicating GMP validation processes. Single use membrane technology is more expensive than other chromatography resins that can be cycled multiple times before discarding (11).
- Kallberg, K., Johansson, H-O. and Bulow, L., “Multimodal chromatography: An efficient tool in downstream processing of proteins,” Biotechnology Journal, 7, 1485–1495 (2012). DOI 10.1002/biot.201200074
- McLaughlin, L.W., “Mixed-mode chromatography of nucleic acids,” Chemical Reviews, 89, 309-319 (1989). DOI: 10.1021/cr00092a003
- Chen, S.W. and Zhang, W., “Current trends and challenges in the downstream purification of bispecific antibodies,” Antibody Therapeutics, 4, 73–88 (2021). DOI: doi:10.1093/abt/tbab007
- Kumar, A. et al., “Design and validation of linkers for site-specific preparation of antibody-drug conjugates carrying out multiple drug copies per cysteine conjugation site,” International Journal of Molecular Sciences, 21, 6882 (2020). DOI: 10.3390/ijms21186882
- Bio-Rad, “Introduction to Multimodal or Mixed-Mode Chromatography.” Available here
- Roberts, J.A. and Carta, G., “Protein adsorption and separation with monomodal and multimodal anion exchange chromatography resins. Part I. Multicomponent adsorption properties and frontal chromatography,” Journal of Chemical Technology and Biotechnology, 97, 3292-3305 (2022). DOI: 10.1002/jctb.7239
- Zhang, K. and Liu, X., “Mixed-mode chromatography in pharmaceutical and biopharmaceutical applications,” Journal of Pharmaceutical and Biomedical Analysis, 128, 73-88 (2016). DOI: 10.1016/j.jpba.2016.05.007
- Fraterman, S. et al., “New Drug Modalities Offer Promise and Peril,” (2023). Available here
- Osuofa, J. et al., “High-capacity multimodal anion-exchange membranes for polishing of therapeutic proteins,” Biotechnology Progress, 37, 1-11 (2021). DOI: 10.1002/btpr.3129
- Poddar, S., Sharmeen, S. and Hage, D.S., “Affinity monolith chromatography: A review of general principles and recent developments,” Electrophoresis, 42, 2577-2598 (2021). DOI: 10.1002/elps.202100163
- Chen. J. et al., “Recent development and application of membrane chromatography,” Analytical and Bioanalytical Chemistry, 415, 45–65 (2023). DOI: 10.1007/s00216-022-04325-8
Market Development Manager for Process Chromatography at Bio-Rad Laboratories