Don’t Break My Strands
CRISPR-engineered cell therapies with multiple gene knockouts could be limited by double-strand breaks
Emily M. Anderson |
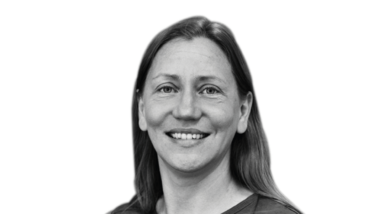
Ex vivo gene editing and cell therapy have the potential to revolutionize how we treat both rare and common diseases. The initial success of cell-based therapeutics for the treatment of blood cancers has set the ball rolling, but challenges, such as the immunosuppressive environment of solid tumors, have yet to be overcome. Another promising technology, CRISPR-Cas-mediated gene editing, is poised to meet these challenges head-on, but not without a few bumps (or breaks) in the road.
CRISPR-Cas9 has a definite advantage over previous forms of gene editing technologies such as meganucleases, engineered zinc finger proteins, or TALENs. Although each of these technologies requires redesign and reconfiguration of a protein to target different sequences of DNA, CRISPR system effector nucleases, such as Cas9, use a small strand of RNA to recognize sequences of DNA – meaning that the same protein can be easily configured to target new DNA sequences by loading a different targeting RNA, known as the CRISPR RNA or crRNA. This new technology democratized the field of gene editing and allowed for high-throughput synthesis and testing of CRISPR editing technology against many human genes in academic and preclinical settings, with an eye to eventual therapeutic applications.
However, we should take a step back and acknowledge that CRISPR-Cas9 and other gene editing technologies have been somewhat overhyped as precision gene editors. In reality, their magic extends only as far as the site-directed cutting of a specific sequence of DNA. This is no small feat, because the human genome contains billions of DNA base pairs, requiring exquisite specificity to target such a needle in a haystack. However, the phenomenon of gene editing comes about through the cell’s repair mechanisms, which are activated as a result of a DNA double-strand break (DSB) introduced by Cas9. In general, the dominant pathways for repair involve either non-homologous end joining (NHEJ) or homology-directed repair using a template. NHEJ induced by a CRISPR-Cas9 cut is often imperfect, leading to small insertions and deletions at the repair site that can disrupt and therefore knock out the gene.
Gene knockout with CRISPR-Cas9 has made it to clinical trials, for instance for the treatment of blood disorders such as sickle cell anemia and beta-thalassemia (1). In this case, much like adoptive T cell therapies for cancer, the edit is made ex vivo and the therapeutic cells are infused back into the patient. However, for cancer immunotherapy, it is likely that more than one gene knockout will be required to augment the properties of the immune cell used for treatment. It is here that the DSBs caused by gene editing could really have an impact.
DSBs are the most cytotoxic lesions naturally occurring in DNA and have the potential to lead to cell death or chromosomal translocations, in which segments of two different chromosomes join aberrantly. Notably, introducing multiple DSBs at once with CRISPR-Cas9 increases the risk of translocations. The number of possible reciprocal balanced translocations (T) increase quadratically with the number of DSBs (N): T=N(N-1) or T=N2-N.
However, translocations can take many forms. They can be classified as balanced, reciprocal translocations, Robertsonian translocations, inversions, de novo translocations, sex chromosome rearrangements, and translocations involving more than two chromosomes. Add to this the possibility that each crRNA used for targeting one site has the potential for off-target effects, meaning that the crRNA could imperfectly pair with other target sites in the genome and cause a break at some frequency (hopefully lower than at the intended target site). The potential for translocations caused by on- and off-target DSBs increases quadratically, so the risks of this strategy mount precipitously. Although most rearrangements have detrimental effects for cellular survival, single events have the potential for a clonal advantage and may result in abnormal cellular proliferation, which could affect the safety profile of the engineered cells or even lead back to cancer.
As an example, a recent clinical trial involving CRISPR-Cas9 knockout of several T cell targets in a TCR therapy model (2) indicated that in general, over many months in several patients with refractory advanced or metastatic cancers, the treatment was safe and well tolerated; however, about four percent of the infused cells contained genome rearrangements. It remains to be seen how improvements in the technology will lead to greater knockout efficiency of multiple genes while reducing this translocation risk for the number and identity of genes targeted.
One way to alleviate the potential danger multiple CRISPR-Cas9 gene knockouts pose to immunotherapy involves gene knockout without introducing a DSB. Base editors have this ability because they make use of a nickase version of Cas9 tethered to a deaminase enzyme. The Cas-targeted deaminase acts on cytosine or adenine bases to convert them to thymine or guanine, respectively, when the deaminated base is repaired via the mismatch repair pathway in the cell. Instead of relying on imperfect NHEJ repair to disrupt gene function, mismatch repair can introduce stop codons or disrupt splice sites, which leads to functional disruption or knockout of a gene (3) without introducing DSBs.
There is little doubt that multiple gene engineering holds great promise to improve the field of cell therapy, but the goal of efficacy balanced with safety requires a cautious approach. Newer generations of gene editing technologies could very well break the “multigene edit barrier” without breaking the system by incurring too many DSBs. Will novel technologies such as base editing take the lead in overcoming this reliance on DSBs and offering safer mutliplex editing of the genome? It is with much anticipation that researchers look to see these developments in the clinic.
- C&EN, “CRISPR gene editing in humans appears safe, and potentially effective” (2020). Available at: https://bit.ly/3wQVXtw.
- EA Stadtmauer et al., “CRISPR-engineered T cells in patients with refractory cancer,” Science, 367, eaba7365 (2020). DOI: 10.1126/science.aba7365.
- BR Webber et al., “Highly efficient multiplex human T cell engineering without double-strand breaks using Cas9 base editors,” Nat Commun, 10, 5222 (2019). DOI:10.1101/482497.