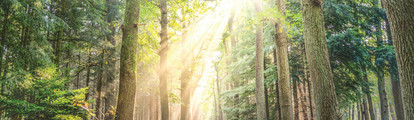
Back to Nature
It’s time for pharma manufacturing to leave behind the highly energy-dependent and inflexible processes of the 20th century and embrace more sustainable and efficient biological approaches. Let’s “biologicalize” manufacturing.
Pharma is undergoing an age of transformation, and by that I mean not just in terms of business models and arguments around pricing, but also manufacturing. The digitalization of manufacturing with “Industry 4.0” is changing the way we think about making drugs, with technologies such as artificial intelligence and continuous manufacturing being intently discussed. But there are also fascinating advances in biology leading to a new transformation that some are calling the biologicalization of manufacturing.
Biologicalization in manufacturing has been defined as, “The use and integration of biological and bio-inspired principles, materials, functions, structures and resources for intelligent and sustainable manufacturing technologies and systems with the aim of achieving their full potential” (1). Bio-inspired chemistries, surfaces and systems have impacted many areas of the global economy, including materials, manufacturing processes, operational technologies and final products.
Manufacturing in the 20th century was – and to a large extent still is – characterized by the use of toxic materials as organic solvents or heavy metals for process chemistries. It often employs dedicated manufacturing facilities and reactors exhibiting process inefficiencies and undesired by-products and waste. The integration of natural and biological principles could result in more sustainable and resource-efficient manufacturing approaches, and more environmentally friendly and energy-efficient products.
Nothing new, yet brand new
Making use of biological reactions is not new – the ancient Egyptians used to convert grain sugars to alcohol when making beer, and brewing still uses biological reactions today. But recent advances in systems and synthetic biology have made it possible to precisely engineer microorganisms to perform more useful and efficient functions. In just one example approach, scientists have genetically engineered classic bacterial and yeast platforms to produce products such as gasoline and polyesters. Many different types of organisms can be considered miniature factories, and the next generation of synthetic biology promises even more pharmaceutically-relevant applications. The genes encoding catabolic or processing functions are being re-engineered to provide entirely new pathways to produce the desired (unnatural) products, for example. Through this, and the careful selection of the appropriate host cell or organism, novel and bioactive molecules can be produced without the need for harsh reaction conditions or environmentally dangerous chemicals.
Biologicalization has also been applied to small-molecule pharma. Examples here include a novel crystallization induced dynamic resolution-based aprepitant synthesis, which eliminates over 80 percent of water, raw materials and waste; the Diels-Alder reaction, providing 100 percent atom economy (2); and a catalytic method of ibuprofen synthesis that nearly doubles atom efficiency and eliminates significant amounts of metal catalyst waste.
But a central problem with using biological systems at manufacturing scale is that they are often slow, inefficient and unstable since they are living systems. But this is improving with the integration of bio-inspired tools with modern “digital” and automated manufacturing schemes. This includes cloud-based data techniques, machine learning, AI and autonomation. Application of the industrial internet of things (IIoT) and model-based control is also changing the face of modern manufacturing. A harmonization of digital manufacturing principles with structures and chemistries from biological systems may define the next generation of manufacturing technology and systems. Klaus Schwab, founder and executive chairman of the World Economic Forum Geneva, famously put it, “the digital revolution […] is characterized by a fusion of technologies that is blurring the lines between the physical, digital, and biological spheres” (3). This transformation will involve sustainable materials and manufacturing processes, resource-efficient products and services, as well as new medical treatments based on the application of both biological mechanisms and 4.0 digital approaches. For example, labile protein biologicals such as Cerezyme and Fabrazyme have, for decades, been produced in perfusion bioreactors culturing animal cells. However, the industry has tended to avoid such procedures because of the lack of efficiency and control required for large-scale biopharmaceutical manufacturing. Today, advances in in-line monitoring, multiplexed analytics and model-based control are providing robust and intensified perfusion processes that are taking over the industry.
We all know cells in our body use enzymes to catalyze the reactions required for life. These enzymes are now being repurposed to produce useful compounds that are difficult, expensive or employ problematic chemicals to make using traditional chemical synthesis methods. Researchers are already stringing together in-vitro enzyme reactions to produce pharmaceutical entities through more environmentally friendly reactions.
Integral to the development and application of these principles is progress in our understanding of biological systems. One of 2018’s Nobel Prize in Chemistry winners was Frances H. Arnold, Professor at the California Institute of Technology, who invented systems for the directed evolution of enzymes, which is now routinely used in the development of tools such as catalysts in manufacturing. This technology also supports other 4.0 goals, such as the environmentally friendly manufacture of renewable fuels and pharmaceuticals (4).
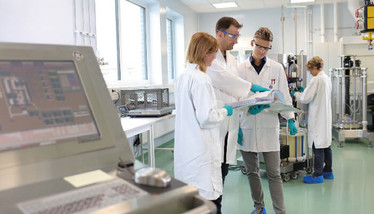
Many highly synthetic and energy demanding manufacturing processes used today actually have biological origins. The observation that wine turns to vinegar led to the so-called German Method of acetic acid manufacturing. Here, an alcohol-containing feed (created by natural fermentation) is percolated through a tower packed with wood shavings seeded with Acetobacter. This process supported the sequential aerobic fermentation of the alcohol by the bacterium, allowing the collection of a solution of acetic acid at the bottom. This was considered a modern and efficient process until it was discovered that it could be generated synthetically. The first published synthetic acetic acid reaction sequence consisted of chlorination of carbon disulfide to carbon tetrachloride, followed by pyrolysis to tetrachloroethylene and then aqueous chlorination to trichloroacetic acid – finally concluding with an electrolytic reduction to acetic acid. Such first-generation highly synthetic and unnatural manufacturing methods, while more productive than the original, are what have led us to the 20th century style of manufacturing that we now understand to be rather unsustainable.
Embracing sustainability
Today we are seeing the biologicalization of the process through many approaches, including the engineering of bacteria to express high levels of new alcohol dehydrogenases – enzymes instrumental in the conversion of ethanal to acetic acid. Such genetic and metabolomic engineering, as well as digital manufacturing-based advances in fermentation technologies, are making acetic acid manufacturing more sustainable, including lower manufacturing energy requirements and the possibility of employing low-cost carbohydrate sources as organic wastes and agricultural residues.
Such approaches also exist for the manufacture of protein, biological and cell therapy products. For example, manufacturing of oligonucleotides for antisense, aptamer and siRNA-based therapeutics previously evolved using solid-phase techniques. Yet, the Caruthers’ phosphoramidite chemistry upon which it is based requires a number of toxic and difficult solvents – and has remained largely unchanged for over 30 years. But solutions employing more water and fewer organic solvents and metal catalysts are becoming more widespread. One illustration of this progress is that acetonitrile and methanol are still popular solvents in much chromatography, but they suffer from several drawbacks from an environmental point of view. Alternative, greener mobile phases employing β-cyclodextrins as mobile phase additives produces a remarkable increase in water compliment for the mobile phases, without loss in the resolution or efficiency of the separations. It’s important to note that the cyclodextrins themselves are prepared by enzymatic treatments of simple starch: commonly, cyclodextrin glycosyltransferase and α-amylase. The manufacturing of RNA vectors, a key component of mRNA-based gene therapy, yields a story similar to acetic acid history. The old cell-based “natural” approach to manufacture yielded a relatively low mRNA yield and required purification from a multitude of contaminants. Newer polymer chemistry-based approaches worked well, but these methods often came with negative characteristics associated with highly synthetic chemistries. For these reasons, we now see bio-inspired, but cell-free aqueous liquid-phase methods most commonly employed. DNA-template directed polymerase (enzyme)-led transcription processes are very efficient and much more sustainable approaches (5).
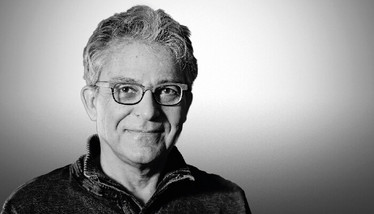
Bill Whitford
In the large-scale production of polypeptides smaller than around 50 amino-acids, classic methods of fermentation of recombinant prokaryotes are generally regarded as inefficient. Direct chemical synthesis is currently a popular means of production, but, here too, the problems are similar to those above. Next-generation digital and continuous fermentation in highly recombinant hosts, engineered immobilized or cross-linked biocatalysts, and site-directed mutagenesis (rationale design) of native enzymes may soon change this. These approaches can demonstrate high specificity and reactivity under mild operational conditions, while reducing the need for stringent equipment requirements, the number of operations performed, energy input required, as well as overall environmental impact.
Illustrating the increased interest in the field, Fraunhofer-Gesellschaft (based in Berlin) sponsored a meeting last June on current initiatives to replace many of pharma’s highly artificial and manual manufacturing approaches with more biomimetic and digitally directed procedures and chemistries (6). This meeting, The Biological Transformation of Manufacturing, brought together experts from around the world to discuss both goals and mechanisms for supporting the increased use of this biotechnology for sustainable growth and innovation. There, we heard pharma-relevant lectures from speakers such as Thomas Schmitz-Rode, Director, Helmholtz Institute of Applied Medical Engineering, RWTH Aachen University, who has developed his thesis on how “control of biological uncertainty in the production process is the key to quality-assured, individualized cellular therapy…” While there have been movements to “go back to nature” for decades (and this is a very prominent trend amongst consumers today), manufacturers today are focusing more on manufacturing efficiency, bio-integrated but novel technologies, and modern digital and nano-techniques. The field is in its infancy, and the tools and approaches are rather diverse and open to much interpretation. Just as a chemical supplier’s final product is actually a pharmaceutical manufacturer’s raw material, it can be difficult to categorize a new bio-integrated technology or bio-inspired material as a means, or consequence, of biologicalization. Nevertheless, biologicalization can apply to what we categorize as a raw material, production means, product technology, and even bio-intelligent systems and organizations.
Whatever the specific chemistries, technologies or applications – “biologicalization” promises to change our concept of manufacturing. Leaving behind the old 20th century concept of highly energy-dependent, inflexible and dangerous chemical-employing processes, and embracing more efficient and biomimetic procedures employing water-based and sustainable chemistries, will enable us to better support our societies in the coming century.
- G Byrne, “Biologicalisation: Biological transformation in manufacturing”, CIRP-JMST, 21, 1-32 (2018).
- H Oikaea, “Nature’s Strategy for Catalyzing Diels-Alder Reaction”, Cell Chem Biol, 21, 429-30 (2016). PMID: 27105277.
- Biospace, “How biotechnology and the fourth industrial revolution could save the world” (2018). Available at: bit.ly/2AarCdO. Last accessed January 8, 2018.
- Nobel Prize, “The Nobel Prize in Chemistry 2018” (2018). Available at: bit.ly/2ydCLtE. Last accessed January 8, 2018.
- X Wu, “Improving acetic acid Production by over-expressing PQQ-ADH in acetobacter pasteurianus”, Front Microbiol, 8, 1713 (2017). PMID: 28932219.
- Omics Online, “Design and production of mRNA-based gene vectors for therapeutic reprogramming of cell fate” (2015). Available at: bit.ly/2OULZ4f. Last accessed January 8, 2018.
Bill Whitford is Strategic Solutions Leader at GE Healthcare.