Cell and Ex Vivo Gene Therapies: A Manufacturing Odyssey
Demand for cell and gene therapies is increasing, but are current manufacturing approaches up to the task? Technology companies are on the case and new solutions are emerging. Here, we explore the key considerations when developing ex vivo gene therapy and cell therapy processes for commercial manufacturing.
George Todorov | | 17 min read | Technology
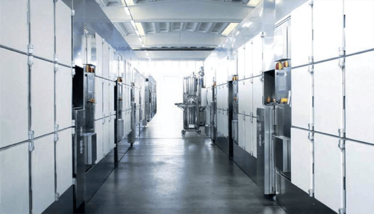
In recent years, ex vivo gene therapies have stirred hope for a curative treatment for B cell malignancies and, in the future, solid tumors. Somatic cell therapies have also been shown to be effective against metastatic prostate cancer and in hematopoietic or immunologic reconstitution therapies.
CAR-T, CAR-NK, and T cell receptor (TCR)-T cell therapies are generated by administering recombinant genetic material that alters the properties of living cells. Genetic alteration of the cells is performed outside the body before the cells are delivered to the patient, so these therapies are classified as ex vivo gene therapies. In contrast, somatic cell therapies are human cells transplanted to repair damaged tissue or cells, and include modalities such as hematopoietic or mesenchymal stem cells and cellular immunotherapies.
Both ex vivo gene therapies and somatic cell therapies have seen clinical success and commercial licensure. Cell immunotherapy products such as CreaVax RCC and Immuncell-LC have been licensed in South Korea since 2007. Dating back to the early 2010s, Dendreon’s Provenge was among the first somatic cell therapies to receive FDA and EMA approval. However, CAR-T cell therapies have taken longer to reach commercialization. Yescarta and Kymriah secured FDA approval in 2018, paving the way for others, such as Tecartus, Breyanzi, and Abecma.
Ex vivo gene therapies and somatic cell therapies come in two flavors: autologous and allogeneic. Autologous cell and ex vivo gene therapies are often considered a safer approach than allogeneic equivalents because there is no risk of graft versus host disease. CAR-T therapies were originally established as autologous products with the need to “scale out” the manufacturing processes to serve a growing market. However, scaling out presents major manufacturing and economic challenges at the commercial level, which has led developers to heavily invest in allogeneic modalities. Allogeneic products can enable scale up of manufacturing processes and “off-the-shelf” solutions that will treat large patient populations, while also lowering cost and reducing manufacturing and supply chain complexity.
Planning for the future
With the number of cell and ex vivo gene therapy products entering the clinic growing exponentially, we must, as an industry, look ahead and plan accordingly so that we can deliver these revolutionary medicines to large patient populations safely and efficiently. Regardless of the specific modality – and the autologous or allogeneic format – cell therapy processes are not standardized and there is significant room for evolution. A number of serious questions remain unanswered:
- How does one efficiently scale out or scale up autologous and allogeneic manufacturing processes to meet growing demand?
- How can an inherently open manual process be converted to a closed and semi or fully automated process?
- What does the evolving regulatory landscape for cell and ex vivo gene therapies push us to anticipate when designing a manufacturing process?
- What do we, as an industry, need to consider when planning to manufacture multiple patient lots and/or multiple cell therapy products under the same roof?
- How can the cost of manufacturing be lowered to make these life-saving therapies more accessible?
The autologous way
The autologous cell therapy industry has rapidly embraced single-use technology and closed processing for GMP manufacturing, but currently available formats for cell processing present logistical challenges for high capacity multi-product and/or multi-client manufacturing. Equipment developers have in recent years provided two contrasting approaches for closed processing of autologous therapies:
i) Modular, single-use equipment that addresses the need of individual processing steps or stages (apheresis, cell isolation, engineering, expansion, and harvest/formulation).
ii) End-to-end equipment with single-use consumables that encapsulates the entire process, following apheresis, in a single instrument.
The hype around CAR-T cell therapies has spurred bioprocess equipment manufacturers into a competitive race to offer modular instrumentation. The result? Plenty of choice for manufacturers. Terumo, Thermo Fisher Scientific, Cytiva, Fresenius Kabi, and others now offer a variety of instruments with parallel functionality, leaving the end-user with several possible configurations and a wide range of processing strategies. Similarly, there are now multiple vendors offering end-to-end solutions in a single system, including Miltenyi, Lonza, Draper, and Cellares. Developers must carefully consider the tradeoffs between housing the process in one system versus multiple specialized instruments.
End-to-end instruments have a small footprint but this approach ties up the whole instrument for 1-2 weeks while a single batch is produced. Separate instruments, on the other hand, can have higher utilization while accommodating multiple batch processing in the same space. This is possible because not all manufacturing steps require the same amount of time to complete. For many processes, the cell expansion operation requires the bulk of the manufacturing time. With separate instruments, facility footprint and capital can be dedicated to the cell expansion equipment to minimize the bottleneck, while other equipment can be limited in quantity and readily shared between batches.
Unfortunately, there is no one right answer; the best configuration is largely dependent on the drug manufacturer’s capacity requirements, process duration, available cleanroom space, and manufacturing model (in-house versus CDMO). Benchtop end-to-end solutions may be a great approach for small clinical programs or quick manufacturing processes and, as throughput demands increase and plans are made for commercial scale-out, it is common to see clients adopt a hybrid approach. For example, clients may perform T cell enrichment, activation, and transduction in a Miltenyi CliniMACS Prodigy, expansion steps in wave reactors, and harvest/wash/formulation in a Cytiva Sefia or similar instrument. In this example, a low number of Prodigy and Sefia instruments are used for the front and tail ends of the process, while an army of wave reactors takes care of parallel batch expansions to increase throughput and allow for parallel processing in the same space. If hybrid and modular processing approaches for autologous manufacturing retain their utility as the industry matures, there will be a significant opportunity to weave in automation. It is also possible to envision robotic systems shuttling and manipulating batches of cells between modular instruments, which would increase efficiency and throughput, while lowering operating costs.
Adding complexity to the maze of end-to-end instrumentation, Lonza offers the Cocoon, which encapsulates the entire process in a single-use cassette format (see Figure 1). It delivers similar capability to the CliniMACS Prodigy, in a much smaller footprint, and can integrate with the Lonza Nucleofector to enable electroporation. Lonza is also developing a Cocoon Tree format that enables a compact scale-out approach by packing a large number of Cocoon pods in a small space, which could be a highly effective solution for high-capacity manufacturing plants or CDMO facilities. When manipulation is required, a motorized system rotates the pods to make them accessible for the operator. Another option, expected to hit the market in 2024, is the Cellares Cell Shuttle – a fully automated end-to-end cell therapy system that uses an industrial robot to move closed cell processing cartridges between unit process operation stations from cell enrichment to formulation. However, the Cell Shuttle does not integrate a fill station, so the user must consider a separate filling solution. The system can execute over 10 workflows simultaneously and, by taking advantage of this format, developers can cue up multiple batches staggered behind one another (see Figure 2).
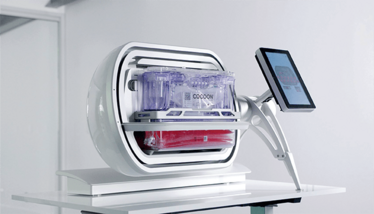
Figure 1. Lonza Cocoon (1).
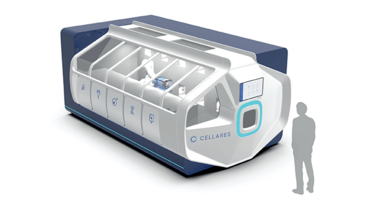
Figure 2. Cellares Cell Shuttle (2).
Closed or open?
Today, there is an abundance of closed processing options for autologous ex vivo gene and cell therapies, all with their own pros and cons, but the majority of cell therapy programs begin R&D and process development in academia with open, manual operations in a biological safety cabinet (BSC). It is a tremendous challenge to rapidly transition a BSC process to closed systems – and the cost of single-use equipment is usually prohibitive to academic labs. However, it is critical to industrialize academic processes prior to technology transfer for GMP manufacturing to avoid program delays, improve product safety, and simplify regulatory review. To help address this gap, developers and academic institutions are investing in collaboration centers that will help bring GMP manufacturing infrastructure and equipment to cell therapy programs developed in academia.
But what about leaving the process open? Sure, this may be an unpopular idea these days, but avoiding a complete overhaul of the original BSC process can accelerate development timelines – if developers have a good approach to maintaining an aseptic environment. In recent years, isolator manufacturers have heavily invested in the ex vivo gene and cell therapy industry to bring these novel processes into well-established Grade A aseptic processing environments. Companies such as ProSys, Comecer, SKAN, Harro Hofliger, OPTIMA, and their partners have delivered innovative isolator configurations that offer end-to-end processing for manual or partially automated manufacturing, and manual or fully automated filling systems for fully closed vials or cryo-bags.
Adopting isolator technology comes with significant upfront investment, which often scares away small companies. However, this knee-jerk aversion to the cost fails to consider the long-term advantages of isolators in commercial manufacturing. Adding to the advantages of operating in an aseptic environment, housing the process in an isolator can realize considerable cost savings during the facility build and operation because this approach enables manufacturing in a Grade C room rather than Grade B. An isolator system may cost several million dollars, but the annual disposable gowning cost for a single technician operating in a Grade B cleanroom can run to ~$30,000, excluding Grade B operator training, qualification, and re-qualification. One year of operation can quickly surpass the cost of an isolator when operating a cell therapy facility of ten or more Grade B suites, to a point where the isolator truly pays for itself in such settings.
Furthermore, modular isolator configurations allow for process and equipment flexibility because they can be configured and strung together with bespoke modules that can evolve with the process (see Figure 3 as an example). This type of configuration allows for independent decontamination of each module, enabling multi-product manufacturing in the same space. Operating the isolator in an assembly line fashion maximizes system utilization as batches progress through processing modules. Though several isolator providers offer similar functionality, some are taking leaps in engineering automation solutions to enable high throughput processing. For example, CO.DON AG is manufacturing their Spherox product in an automated facility featuring Comecer’s FLEXYCULT mobile incubation system for docking incubators that are shuttled between isolators and a CNC area using a robotic handler running in a central spine corridor (see Figure 4). Innovations such as modular processing isolators and the FLEXYCULT enable processing of a large number of autologous batches in a small number of isolators, and allow us to dispel the notion that legacy manual processes cannot be scaled out.
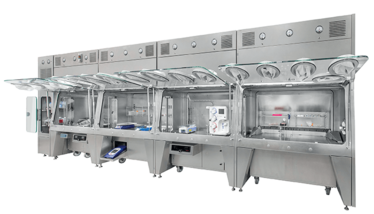
Figure 3. Modular isolator configuration, courtesy of SKAN (3)
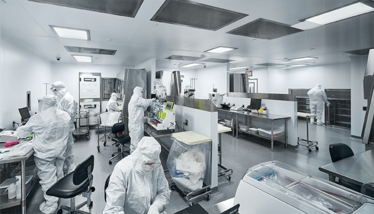
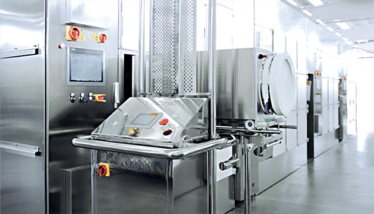
Figure 4. FLEXYCULT system with incubator handler in CNC, courtesy of COMECER (4). Grade B image supplied by IPS.
It is important to note that all of the approaches to multi-product manufacturing described above are closely monitored by regulators, and are acceptable provided adequate measures are taken to prevent cross-contamination and mix-up of materials. Chain of identity is paramount for autologous products; hence, sponsor companies must have established and validated systems to track the donor material and engineered cells throughout each step of the process, subsequent sampling, storage, and shipment. It is only acceptable to house more than one batch or product in the same space if using closed and contained systems. Regardless of whether one uses closed single-use equipment or isolators, the EU Guide on GMP specific to ATMPs calls for 100 percent air exhaustion when using more than one viral vector for engineering ex vivo gene therapies in the same room.
Allogeneic considerations
While autologous therapies are often considered the faster route to securing life-saving treatment, such a generalization fails to consider the supplier’s total batch capacity and the point at which batch production can actually begin. Allogeneic therapeutics can circumvent the nightmare scenario of a patient dying before an autologous batch production is complete or even initiated by providing an off-the-shelf alternative that could potentially help thousands of patients per batch. Autologous and allogeneic ex vivo gene therapies and cell therapies share many process elements, and though the cell expansion and harvest may vastly differ in scale, they have common scientific principles. These similarities present some advantages to developers and CDMOs looking to transition from autologous to allogeneic – or wanting to house both modalities under one roof. That said, here are few key differences to bear in mind:
- A healthy donor typically provides the starting material.
- Tissue typing should be carefully matched with receiving patients to avoid host rejection or the need for immunosuppression.
- Isolated immune cells or stem cells are banked prior to initiating the manufacturing process, akin to a master cell bank that is used for biologics manufacturing.
- Allogeneic cell therapies are expanded to a much larger scale.
Regardless of the therapeutic modality (CAR-T, stem cell, cellular immunotherapy), allogeneic processes require scaling up rather than scaling out – taking the early-stage process and increasing the output of product using larger equipment sizes or volumes to generate enough product to treat a larger patient population. However, these therapies are generated with primary human cells that have a finite population doubling, so the feasible culture scale-up cannot currently reach the volumes of biologics. Combining scaling up and scaling out together could be a useful approach to address limited population doubling. For example, scale up to 50 L or 200 L could then be scaled out to multiple 50 L or 200 L reactors. Culture intensification is also highly desirable to maximize the cell density of allogeneic and autologous batches, which is why some developers are using perfusion to increase the output of both modalities.
The scale up challenge
When planning for scale up, care needs to be taken to select a scalable bioreactor platform and to provide adequate space, utility connection sizes, and locations. In many cases, ceiling-based utility panels are used for flexible equipment location and size. In the case of specific workstations or areas, planning for increased equipment size or relocation of equipment should be done ahead of time to ensure that operation can be maintained after the changes are implemented. Culture scale up principles from biologics manufacturing are applicable and transferrable, including maintaining a power-volume ratio or constant oxygen mass transfer coefficient to ensure autologous cells are exposed to similar conditions across bioreactor scales. Human stem cells and primary cells are much more sensitive to shear stress than immortalized cell lines, so alternatives to stir-tank reactors may need to be considered. Besides wave reactor technology, some manufacturers, such as Kuhner and PBS Bio, offer scalable low-shear single-use reactor systems of up to 500 L that can be used for suspension or microcarrier cultures. Harvesting and formulating allogeneic products will require appropriately scaled single-use centrifugation systems with buffer exchange functionality. The Sartorius kSep platform and the UniFuge line of instruments from Carr Separation Technologies both offer flexibility in scale, excellent recovery and viability.
The final fill, inspection, and labeling steps present a looming challenge to allogeneic processes as they grow in scale. After the point of DMSO (or other cryopreservative) addition, there is a narrow window of 1-2 hours during which the product must be filled, inspected, and labeled so that the cryopreservation cycle can be started in time to avoid damage to the cells. It is therefore imperative to get a head start on studying and designing these final steps to avoid costly complications during a facility design or late stage clinical studies. Automated vial filler providers, such as Aseptic Technologies and Flexicon, offer sophisticated and customizable systems that can be integrated into isolators to maintain an aseptic environment (see Figure 5A). Processes terminating in a bag fill rather than vials can also be automated and scaled. Innovators such as Single Use Support now provide aseptic filling systems that feature a single-use disposable fluid path and can simultaneously fill multiple single-use bags to accommodate a wide range of batch sizes (see Figure 5B). Selecting an isolator and/or automated filler are important steps, but they must not be done in a vacuum. Understanding the throughput requirement, batch size and timing of filled vial nests or batches of bags exiting the filling chamber is critical to informing the strategy for labeling and inspection.
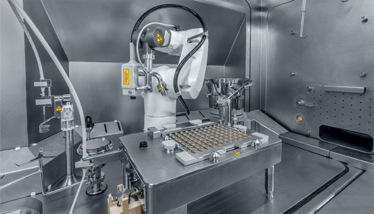
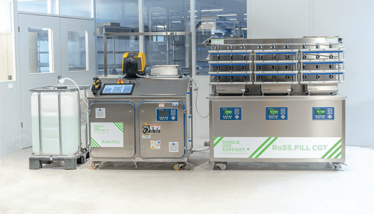
Figure 5. Crystal L1 robotic vial fill line (A) and Single Use Support ROSS.FILL CGT bag filler (B) (5, 6).
Manual inspection is time-consuming and, as batch sizes increase, one must plan for multiple inspection stations, adequate space, and enough personnel. Semi-automated or automated inspection solutions are available from various suppliers, including Korber Pharma, Antares Vision, and Brevetti. This kind of instrumentation was originally designed for large-scale pharmaceutical inspection; however, scaled down versions are now available to serve the cell and gene therapy industry. Analogous to the isolator paradigm discussed above, automated inspection equipment is a costly investment but can decrease processing time, staffing needs, gowning costs, and the required cleanroom footprint. Also, keep in mind the fact that manual or automated visual inspection must satisfy USP 788, 790 and 1790 guidelines, and implementing automated inspection requires lengthy validation studies that must be executed at the manufacturing site.
Last but not least, labeling can be manual or automated, but both the timing and location for this operation must be considered. Labeling is typically done in a Grade D or CNC environment, but some cell therapy developers consider performing this function in the filling suite to avoid wasting valuable time in moving the product to another area ahead of cryopreservation. Performing labeling in the filling suite raises the concern of introducing particulates in a Grade C environment and one must properly package and sanitize incoming labels, but alternative strategies are now available, such as laser-etching QR codes and product information on the vials prior to the fill or applying pre-printed label sleeves following cryopreservation, to alleviate the fill/finish timing constraint.
The road ahead
Autologous and allogeneic ex vivo gene therapies have significant process overlap, including cell isolation, activation, engineering, initial expansion, formulation, and the need for cold chain. For example, cell isolation, activation, and expansion procedures take advantage of antibody-conjugated paramagnetic beads to capture the correct cell type or mimic interactions that trigger activation and cell expansion. A magnetic field is applied to isolate cells that will be engineered into autologous or allogeneic products, or to remove magnetic beads from the culture after processing. Biodegradable paramagnetic beads are also readily available and developers must weigh the benefits of eliminating the de-beading step against the results obtained with different bead products and the risk of introducing residual impurities.
Engineering ex vivo gene therapies to express a CAR gene is predominantly carried out by transduction with a lentivirus or other retroviral vector. Viral transduction is completely expandable to allogeneic process scales, but one must consider that retroviruses are typically handled in BSL2 environments, which require proper measures and facility design for biocontainment and segregation. Aside from the safety concern, the cost and timeframe required to produce GMP-grade viral vectors are significant so it’s worth considering alternative options. Electroporation methods use an electric field which temporarily permeabilizes the cell membrane, allowing for uptake of DNA into the cell. This technology started out as a cuvette-based benchtop format but has expanded into a scalable single use format offered by companies such as MaxCyte and Lonza, enabling its use in large scale allogeneic processes. As the industry continues to mature, expect to see wider adoption of electroporation instrumentation in cell therapy and viral vector manufacturing.
Looking toward the future of these life-saving therapies, raw material suppliers, equipment manufacturers, architecture and engineering firms, and drug developers all need to coordinate efforts aimed at reducing manufacturing cost and expediting delivery to patients in dire need. Process closure, end-end solutions, automation, and de-classifying manufacturing space are all strides in the right direction on our journey to make these therapies more accessible. To add more manufacturing capacity, the industry is looking beyond scaling out autologous and scaling up allogeneic processes. But one can envision a future where equipment innovators and regulators come together to enable decentralized end-end bedside autologous cell therapy manufacturing across a wider network of hospital environments.
- Lonza, “Cocoon platform.” Available at https://bit.ly/3F9JKHI
- Cellares. Available at https://bit.ly/3ik2ScJ
- Skan, “Cell and gene therapy ATMPs.” Available at https://bit.ly/3VBJ2IB
- Comecer, “CO.DON. Cell culture isolators and Flexycult incubation system from advanced therapy labs.” Available at https://bit.ly/3EM0x23
- Aseptic Technologies, “Crystal filling lines.” Available at https://bit.ly/3AR389B
- Single Use Support, “Cell and gene therapies filling system – ROSS.Fill CGT.” Available at https://bit.ly/3uaJj9q
Cell & Gene Therapy Process Expert at IPS – Integrated Project Services LLC