Gene Therapy: The Rocky Road to Success
A rapid review of gene therapy’s rise – and a quick glimpse into the future
Alexandra Brinkman | | Longer Read
The manipulation of genes to treat disease once seemed only to be the basis for an average science fiction novel. And if your story restored an individual’s vision by using a virus to replace a mutant version of a gene with a healthy one, you might have a best seller on your hands. But we all now know that this is not fiction; it is a description of Luxturna, the first FDA-approved gene therapy for an inherited retinal disease caused by mutations in both copies of the RPE65 gene.
The concept of gene therapy was officially established back in 1972. And although gene therapy is considered a relatively new area of therapy, the field can be dated even further back to 1928, when Frederick Griffith first described the transforming principle. By 1968, Rogers and Pfuderer performed the first proof-of-concept viral mediated gene transfer. Fast-forwarding to 2003, China became the first country to approve a gene therapy for clinical use. By 2009, the first successful phase III trial of a gene therapy had occurred in the EU and in 2012, the EMA recommended the first gene therapy product for approval. Following on from this success, the FDA approved Luxturna, the first gene therapy for an inherited disease, in 2017 (1).
However, the road for gene therapy has not been smooth. Here, I look at genetically driven non-oncology indications, based on data from Beacon Targeted Therapies, highlighting the challenges and successes of the field.
Setbacks and successes
According to the Gartner hype cycle, a graphical representation depicting the maturity of novel technologies, gene therapy reached its peak of inflated expectation in the mid-1990s. This inflated expectation was paralleled by a rapid rise in clinical trial activity and the publication of early proof-of-concept studies for genetic conditions, such as adenosine-deaminase deficiency (ADA-SCID). It had been clear from the start that gene therapy had potential, however, focus needed to be given to the basic science of gene transfer and its respective technologies. Following this peak of excitement, the field descended into the “trough of disillusionment” following the death of Jesse Gelsinger during a clinical trial for ornithine transcarbamylase (OTC) deficiency (2). Consequently, concerns over the future of gene therapy were raised – and further complicated by an increasing awareness of the challenges that would be encountered, including vector-induced immune response (immunogenicity) and decreased financial investment. To overcome one (possibly both) of these challenges, researchers have focused on improving their understanding of disease pathophysiology to develop safer and more efficient vectors.
The research has resulted in recent clinical successes for inherited orphan diseases from the treatment of Leber’s congenital amaurosis to the EMA approving Glybera in 2012 for lipoprotein lipase deficiency (3). These successes are driving the field up what is known as the “slope of enlightenment” and into a “plateau of productivity.” As a result, we’ve seen greater innovation in strategies addressing editing and vector technology, and the creation of more biotechnology companies dedicated to gene therapy development – backed with substantial financial investment.
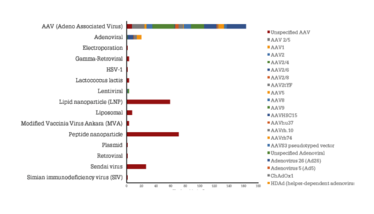
Figure 1. Vector distribution based on the number of active drugs within gene therapy. Source: Beacon Target Therapies, October 2020.
We should not become blinded by the success gene therapy is having. The field is young and dynamic, but still faces challenges around transduction efficiency, clinical trial endpoints, and, most significantly, immunogenicity. As AAV vectors can be administered directly to the patient, the likelihood of a host immune response is high. Additionally, any pre-existing responses to the wild-type virus that the vector is engineered from, or the transgene product itself, can interfere with therapeutic efficacy if not identified and managed optimally. It is, therefore, important for the field to gain a better understanding of the different viral and non-viral platforms used so that immunogenicity can be minimized. Currently, the AAV vector is the most frequently used delivery system based on the number of active drugs, but also contains the greatest immunogenicity challenges (see Figure 1). Outside of AAV vectors, there are further novel vectors being created to help evade the immune response – and the clear innovation occurring in the non-viral delivery space can help to overcome the immunogenicity problems seen with AAV vectors (4).
A key challenge inherent to immunogenicity is our ability to measure and predict the immune response. A lack of standardization of regulatory protocols for assays and immune prediction levels – or, put another way, what level of an immune response is acceptable – have created problems. Patient stratification, as defined by the amount of neutralizing antibodies present, can be one alternative to help mitigate immunogenicity. It can then be decided which patients are enrolled onto trials as part of the inclusion criteria (for example, anti-adenovirus antibodies). Another strategy being used to help prevent immunogenicity is immunosuppression/patient conditioning. Though the strategy reduces unwanted immune responses to the gene therapy, there are still questions around its use; specifically, are immunosuppressants suitable for long term use? Furthermore, efficacy of the gene therapy can potentially be compromised by use of immunosuppressants. Standardization of immunosuppression protocols, such as inclusion/exclusion of use, would need to be better defined. And yet, though these are hurdles to be addressed, they have not stopped gene therapy from being validated as a therapeutic approach – and that is reflected in the present-day landscape.
Changing approaches
The discovery of RNAi enabled a shift from only focusing on gene augmentation and replacement to also focusing on downregulation of genes – namely, mutant variants that can result in a therapeutic effect on disease pathogenesis. This shift is reflected by recent approvals, such as Givosiran in 2019 and Patisiranin in 2018, as well as the increasing number of RNAi clinical trials over the last decade (CAGR of 28 percent) for a variety of disease indications (see Figure 2). Some of the first clinical therapies using small‐interfering RNA (siRNA) were for diseases of the liver, as siRNA sequences were initially developed to target hepatocytes. Examples of this type of therapy include treatments for transthyretin‐mediated amyloidosis and complement‐mediated diseases (5).
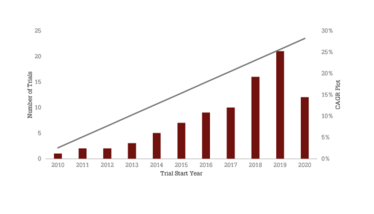
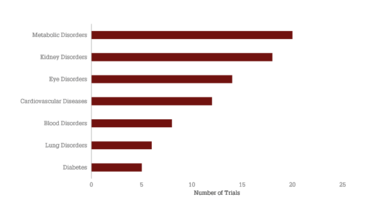
Figure 2. Clinical growth and disease variation within RNAi therapies. A) The number of trials evaluating RNAi therapies initiated per year since 2010 and the compound annual growth rate (CAGR) of 28 percent. B) Distribution of trials across disease indications, with at least five clinical trials using RNAi therapies. Source: Beacon Targeted Therapies, October 2020.
In recent years, gene editing has also taken off. Gene editing tools offer a more elegant and precise method of treating genetic diseases. There have been efforts on this front through ex vivo homologous recombination, TALENS, and Zinc Finger Nucleases (ZFNs). However, clustered regularly interspersed short palindromic repeats (CRISPR)/Crispr‐associated protein 9 (Cas9) has become the editing technology of choice – transforming biomedical science.
CRISPR/Cas9 started receiving attention in 2012 as an editing technique, and the first clinical trials were initiated in 2017 (see Figure 3). The tool was pioneered by Emmanuelle Charpentier and Jennifer Doudna, who received the 2020 Nobel Prize in Chemistry. Proprietary editing technologies are now rapidly being developed across the industry; for example, UniQure’s miQURE silencing technology and CRISPR/OMNI.
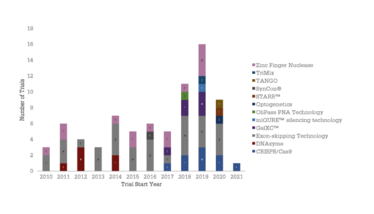
Figure 3. Number of clinical trials initiated each year in the last decade versus editing technology. Source: Beacon Targeted Therapies, October 2020.
And the future?
Although there have been setbacks, the ultimate success of gene therapy has been driven by improvements in both nonviral and viral vectors. Though the majority of assets still use viral vectors, the increasing diversity of the field is reflected in the number of nonviral assets (46 in total) that have entered the clinic for both ex vivo and in vivo gene therapy. Some of these technologies include vectors, such as cationic lipids, plasmids, and peptide nanoparticles.
Based on analysis from Beacon Gene Therapy, 88 trials are expected to be completed in 2021, with 70 trials in 2022. Consequently, an influx of data is predicted in the coming years. Looking at the number of phase III trials expected to be completed over the next five years, we can expect to see new approvals for both classical gene therapy and RNA-based approaches. Alnylam Pharmaceuticals is leading the way with three assets in phase III, but it will be exciting to see who will ultimately win the race for the next approval (see Figure 4).
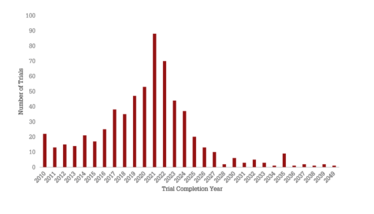
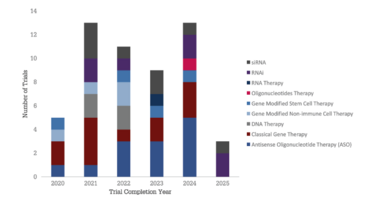
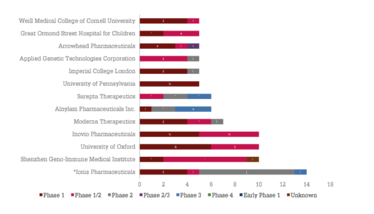
Figure 4: The future of gene therapy. A) number of gene therapy trials completed versus completion year. B) Distribution of phase III trials coming to completion in the next five years, broken down by completion year and therapeutic class. C) Developers with at least five active gene therapy assets broken down by total number of therapies and highest phase of development.
*Ionis Pharmaceuticals has 5 of its 14 assets in co-development with Biogen (4 in phase I and 1 in phase I/II). Source: Beacon Targeted Therapies, October 2020.
According to the ARM 2020 report (6),this year (even taking into consideration COVID-19), is on track to be a record-breaking year for regenerative medicine and advanced therapy financings. Therapeutic developers raised more in the first half of 2020 than in all of 2019 (US$10.7 billion in the first half of the fiscal year 2020 versus $9.8 billion total in 2019). This year will likely provide the best year on record for cell and gene therapy financings ($13.5 billion total), with developers having already raised nearly 80 percent of the full-year total seen in cell and gene therapy financings in 2018. Investors will no doubt appreciate the robust pipeline that each of the gene therapy therapeutic classes offers; however, it is important to appreciate that, due to the immaturity of the field, there are a significant number of companies at the preclinical stage – and there will be both significant winners and losers.
It is also important to note the impact that COVID-19 is still having on the field. The increased number of INDs filed within cell and gene therapy, and a shift in focus towards COVID-19 therapeutics and vaccines, has placed an additional burden on the FDA and led to delays. For example, Sarepta Therapeutics’ asset SRP-9001 for Duchenne muscular dystrophy (DMD) has seen a delay in its clinical trial. While giving late notice, the FDA has requested an additional potency assay for the release of SRP-9001 prior to dosing, resulting in trial delay. During these unprecedented times, it is likely that we will see more trials delayed while the FDA tries to keep the pace with both the increasing number of gene therapy IND applications and the impact of COVID-19 on trial and drug development.
Undoubtedly, the path for gene therapies has been rocky. The field has seen significant setbacks, from understanding disease pathology to safety and immunogenicity concerns, but these hurdles have pushed the field to pursue improved technologies, and the variety of delivery and editing technologies available today offers a perspective on how challenging it is to tackle certain diseases. With several assets already successfully approved and more seeking approval, the approach of the field has been validated; next, we expect to see a domino effect of approvals driven by the increased influx of data, IND filings, and new technologies being implemented.
- AM Keeler, MK ElMallah, TR Flotte, “Gene Therapy 2017: Progress and Future Directions,” Clin. Transl. Sci., 10, 242-248 (2017). PMID: 28383804
- Raper, S. et al., “ Fatal systemic inflammatory response syndrome in a ornithine transcarbamylase deficient patient following adenoviral gene transfer” (2003). Available at: https://bit.ly/3kunJpm
- Bryant, L. et al., “Lessons learned from the clinical development and market authorization of Glybera”.(2013). Available at https://bit.ly/2UJLqQd
- F Mingozzi, KA High, “Immune responses to AAV vectors: overcoming barriers to successful gene therapy,” Blood, 122, 23-36 (2013). PMID: 23596044
- J Baruteau et al., “Gene therapy for monogenic liver diseases: clinical successes, current challenges and future prospects,” J. Inherit. Metab. Dis., 40, 497-517 (2017). PMID: 28567541
- Alliance for Regenerative Medicine, “Innovation in the Time of COVID-19” (2020). Available at https://bit.ly/3ozzLkq.