mAbs: Not so Sweet
How fucosylation-deficient CHO cell hosts can help enhance the potency of monoclonal antibody-based biotherapeutics
Neha Mishra, Jesus Zurdo | | 9 min read | Practical
Early on, one of the central challenges in mAb production was low product titer – but this has since been overcome by advances in cell line development and substantial improvements in cell-specific productivity, and further driven by broader progress in industrial bioproduction technology. Bioprocess optimization, generally achieved via optimization of media and culturing conditions (temperature, speed, etc.), has led to significant improvements in product titers and performance of the host cells. Meanwhile, the ever-increasing demand to develop and manufacture mAbs has led to heavy investment in R&D programs focusing on product quality and consistency.
Approval of biotherapeutics for human use requires the definition and control of a number of critical quality attributes (CQAs) which are key to performance and safety. For mAbs, the presence and type of post-translational modifications (PTMs), such as glycosylation, is a good example (2). Glycosylation is an enzymatic process involving the addition of oligosaccharide structures to specific amino acid sites of polypeptides to form glycoproteins. This non-template based process occurs within the endoplasmic reticulum (ER) and Golgi as the protein transits through the cell before secretion or translocation. There are many forms of glycosylation, but the two most common types are N- and O-linked glycosylation:
- In N-linked glycosylation, oligosaccharides are attached to the amide nitrogen of an asparagine (Asn) residue in a consensus sequence Asn-X-Ser/Thr where X is any amino acid except proline.
- In O-linked glycosylation, oligosaccharides are attached to the oxygen atom of hydroxyl groups of amino acids such as serine (Ser), threonine (Thr) or tyrosine (Tyr).
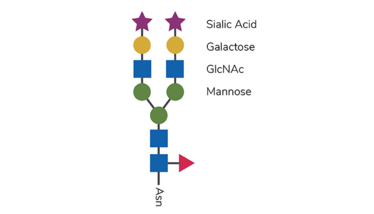
Figure 1. Glycan core structure
The glycan core structure (see Figure 1) presented by antibodies contains N-acetylglucosamine (GlcNAc) and mannose upon which other sugar residues, such as galactose, sialic acid and fucose, are added.
Why is glycosylation so important in proteins?
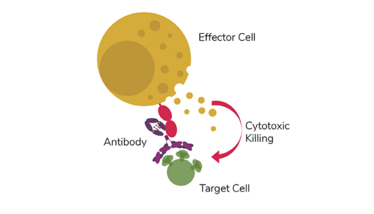
Figure 2. ADCC mediated by effector cells: ADCC response on a target cell via CD16 receptor on an effector cell, triggered with the help of a mAb (figure adapted).
Approximately 70 percent of mammalian proteins are glycoproteins with N-linked glycans, which often confer specific properties to the polypeptide chain. Variation in N-glycosylation of therapeutics can have a significant impact in protein folding, stability, pharmacokinetics, immunogenicity, or even mode of action (2,3). This impact is particularly relevant for mAbs, where variability in the N-glycan structures present in the CH2 domain determines, amongst other things, cell-mediated responses, including antibody-dependent cell cytotoxicity (ADCC) and complement dependent cytotoxicity (CDC).
Given the influence of specific glycans on the therapeutic effect of biologics, the control of glycosylation profiles in biopharmaceuticals, is a highly important topic.
ADCC responses are mediated by the FcγRIIIa (CD16) receptor expressed primarily by natural killer (NK) cells (also known as effector cells). Antibodies recognizing specific ligands on a “target-cell” surface can activate NK cells through the interaction between the Fc region of the antibody and the FcγRIIIa receptor of an NK cell, resulting in release of cytotoxic agents that ultimately eliminate the target cell (see Figure 2). The magnitude of the ADCC response is dependent on the affinity between the FcγRIIIa receptor and antibodies (4). Structural studies have revealed that the presence of fucose on the core glycan structure on IgG1-Fc reduces binding affinity of the IgG1 to FcγRIIIa receptors (5). Therefore, the removal of core fucose in glycan structures of antibodies – known as afucoslylation – is a particularly important strategy in oncology therapeutics.
Advantages of afucosylated antibodies include:
- Effective ADCC responses against tumors exhibiting low antigen-expressing levels. This can be relevant for cancer therapeutics, such as Rituximab, which has been shown to be less effective against lymphomas with reduced CD20 expression (6). The ability of afucosylated mAbs to elicit ADCC responses against cells with low antigen expression levels opens the door to more effective therapeutic approaches against currently unsuitable oncology targets.
- Reduced competition from serum IgGs in binding (and activating) FcγRIIIa receptors. Evidence in clinical settings has shown that therapeutic antibodies can be inhibited by circulating IgG competing for FcγRIIIa receptor binding (7). Higher levels of therapeutic antibodies are therefore required to overcome this competition, which can introduce complications and undesirable side-effects. The use of afucosylated antibodies can reduce such competition by increasing the binding affinity to FcγRIIIa receptors.
By addressing these two factors, afucosylated antibodies could have a significant impact in increasing the potency of biopharmaceuticals, expanding their therapeutic window, and potentially reducing undesirable side-effects and complications associated with treatment, due to the lower doses required to elicit a physiological effect.
The use of glycoengineered mAbs is not restricted to oncology therapies. Complement-dependent cytotoxicity (CDC) is also affected by the glycosylation pattern; antibodies exhibiting low or no galactose and high mannose show a decreased binding to complement component 1q (C1q) complex, leading to a reduced CDC response. Additionally, highly sialylated antibodies can mediate anti-inflammatory responses in autoimmune diseases (8).
Given the importance of glycosylation on effector functions that are mediated by therapeutic antibodies and Fc-fusion biotherapeutics, host cell lines used to express such products can be engineered to produce selective glycoforms that can, in turn, modulate their specific biological activity.
The right tool for the job…
Chinese hamster ovary (CHO) cells have been used for biologics production since the approval of t-PA in 1987. CHO cells can produce human-like PTMs and are robust systems capable of adapting efficiently to different culture conditions, including serum-free media. Importantly, CHO cells are less prone to being infected by human viruses. Recent advances in bioprocess engineering have dramatically increased the performance of these cells and the yields typically obtained in bioproduction (9).
CHO cells usually produce high proportions of fucosylated mAbs, impacting the biological activity of antibody therapeutics they express. Equally, as stated above, other glycan modifications can drastically influence the effector function of mAbs. Therefore, there is great potential in the modification of the glycosylation pathways of CHO cells to generate therapeutics with improved properties. For this, the use of next-generation genome editing tools can offer an effective tool to engineer expression hosts able to produce therapeutics with specific characteristics (10).
There has been a growing interest in controlling the glycan composition of therapeutic proteins, particularly to generate more efficacious therapies by eliminating the fucose content of mAbs. To enrich the proportion of afucosylated antibodies in the final product, several strategies have been explored: (i) control of cell host (CHO primarily) metabolism during cell culture conditions, (ii) inhibitors targeting fucosyltransferase or other fucosylation enzymes, (iii) expression of enzymes to deviate metabolism, reducing available fucose in the cells, and (iv) use of RNAi to repress or reduce transcription of key fucosylation enzymes, amongst others.
However, glycan composition is highly sensitive to external conditions, product, and overall behaviour of cells in culture. Consequently, this creates a problem for developers on two fronts: i) most of these technologies make it virtually impossible to generate therapeutic preparations with 0 percent or 100 percent of their molecules containing a given glycan composition (8), and ii) batch-to-batch variability observed in bioproduction is intrinsically inherent to the nature of the cell culture control systems – and can have significant consequences in drug potency and safety. The latter is particularly acute because potency cannot be simply traced to dose anymore and batch-to-batch variations in glycan composition can have a substantial impact in drug potency. This places additional stresses on manufacturing and quality control that are very difficult to address.
Therefore, there is great potential in the modification of the glycosylation pathways of CHO cells to generate therapeutics with improved properties. In this regard, next-generation genome editing tools can help engineer expression hosts able to produce therapeutics with specific characteristics (10).
When it comes to fucose, one obvious answer lies in engineering host variants that lack the ability to incorporate a fucose molecule in the glycan structure (11). In these types of systems, it is possible to use a functional knockout of a fucosyltransferase gene to inactivate the fucosylation pathway in the cells. Antibodies expressed from these cell lines contain glycans that are devoid of the core fucose as shown by glycan analysis, where 0 percent of fucose is detected. In comparison, mAbs produced from the wild-type parental cell line contain up to around 90 percent of fucosylated glycans (see Figure 3). Afucosylated model antibodies exhibit markedly higher efficacy in eliciting an ADCC response than their fucosylated counterparts when faced with target cells with low antigen-expressing cells and in the presence of NK cells with FcγRIIIa receptor polymorphisms that are known to decrease ADCC functionality (12).
Two glycoengineered mAbs lacking fucose, anti-CCR4 mogamulizumab and anti-CD20 obinutuzumab, have been approved for therapeutic use in 2012 and 2013, respectively (both produced in genetically modified CHO cells). Many more glycoengineered mAbs lacking fucosylation are currently in development in areas as diverse as oncology and infectious diseases (8).
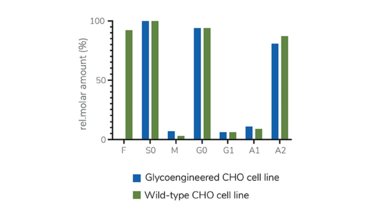
Figure 3. N-Glycan structures detected on trastuzumab (TTZ) control mAb expressed in a wild- type and glycoengineered CHO cell line detected by HILIC-HPLC. TTZ produced in the glycoengineered cell line shows complete removal of fucose from the glycoprotein (data from PerkinElmer’s Horizon Discovery).
Understanding glycobiology
As outlined above, glycan composition is well known to modulate the biological activities of antibodies in our bodies – from regulating half-life to eliciting ADCC or CDC immune responses. Typically, these functions are mediated via endogenous Fc receptors present in different cell types and tissues and influenced by their relative affinity for different Fc architectures (including different amino-acid and sugar compositions).
Glycobiology is, therefore, emerging as an important discipline in the design of more effective biotherapeutics, particularly by modulating effector function in the case of IgG molecules. As we’ve also highlighted, gene editing technologies can be used to engineer host cell lines able to produce afucosylated therapeutic antibodies to enhance ADCC response; indeed, antibodies lacking fucose in their Fc glycan show up to 50-fold increased binding affinity to FcγIIIa receptors of NK cells mediating effector ADCC responses (12, 13). The absence of fucose residue also compensates for the differences in effector function activities across human populations with different polymorphisms in position 158 of the FcγIIIa receptor. More broadly, afucosylated antibodies have shown improved patient responses and outcomes, irrespective of the amino acid present at such a position (13). And this adaptive immune response has much wider applications beyond the development of treatments for oncology, opening the door to applications in a wide range of conditions where better control over ADCC effector function activity is desirable.
The development of antibodies with enhanced ADCC activity has also been increasingly explored in the treatment of infectious diseases, particularly viral infections; there is a growing body of evidence supporting the use of cytotoxic mechanisms of action to control the spread of infection within patients affected by a given virus. This approach has been successfully assessed against a number of different infections, including Ebola virus, human immunodeficiency virus (HIV), respiratory syncytial virus (RSV), and influenza (14).
In short, genetically modified CHO cells can be used to produce afucosylated antibodies with enhanced ADCC activity, which can drive the development of more effective treatments in oncology, infectious diseases, and autoimmune disorders, while offering greater control over product quality and potency.
- G Walsh, Nat. Biotechnol., 36, 1136–1145 (2018). DOI: 10.1038/nbt.4305
- D Reusch, ML Tejada, Glycobiology, 25, 1325–1334 (2015). DOI: 10.1093/glycob/cwv065
- DN Hebert, SC Garman, M Molinari, Trends Cell Biol., 15, 364–370 (2005). DOI: 10.1016/j.tcb.2005.05.007
- UJE Seidel, P Schlegel, P Lang, Front. Immunol., 4, 1–8 (2013). DOI: 10.3389/fimmu.2013.00076
- C Ferrara et al., Proc. Natl. Acad. Sci. U. S. A., 108, 12669–12674 (2011). DOI: 10.1073/pnas.1108455108
- MR Smith, Oncogene, 22, 7359–7368 (2003). DOI: 10.1038/sj.onc.1206939
- S Preithner et al., G. Mol. Immunol., 43, 1183–1193 (2006). DOI: 10.1016/j.molimm.2005.07.010
- R Mastrangeli, W Palinsky, H Bierau et al., Glycobiology, 29, 199-210 (2019). DOI: 10.1093/glycob/cwy092
- KP Jayapal et al., Chem. Eng. Prog., 103, 40–47 (2007).
- J Zurdo, A Gough, D Cougot, IBI, 3, 44-47 (2020).
- N Yamane-Ohnuki et al., Biotechnol. Bioeng., 87, 614–622 (2004). DOI: 10.1002/bit.20151
- N Yamane-Ohnuki, M Satoh, MAbs, 1, 230-236 (2009). DOI: 10.4161/mabs.1.3.8328
- SD Liu et al., Cancer Immunol. Res., 3, 173–183 (2015). DOI: 10.1158/2326-6066.CIR-14-0125
- G Salazar et al., npj Vaccines, 2, 19 (2017). DOI: 10.1038/s41541-017-0019-3
Senior Scientist Bioproduction
Global Head Cell, Gene Therapy & Bioproduction at PerkinElmer's Horizon Discovery