Nanotechnology Versus Cancer
With only one approved drug and many failures, the cancer vaccine field has lost some of its shine. Could nanotechnology brighten its prospects?
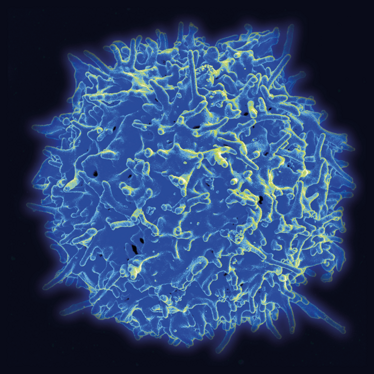
We need a new type of weapon in the war on cancer. Chemotherapy, the backbone of cancer treatment, often brings initial success but cancers are notorious for relapsing in ever more aggressive and resistant forms. Traditional chemotherapies do not discriminate. They attack all rapidly dividing cells and are plagued by a narrow therapeutic index, numerous side effects, and high likelihood of resistance (1). Newer, molecularly targeted agents sidestep many of these problems, but often require lifelong treatment. And because these agents are relatively new, their long-term efficacy and safety are yet to be determined, and it is likely that we will see resistance develop over time.
Harnessing the immune system to fight cancer has become the Holy Grail of oncology. The potential is obvious – by using the body’s own defenses, we could in theory avoid the problems of current therapy and provide new options for cancers that would otherwise be untreatable. Indeed, the journal Science named “immunotherapy of cancer” as its 2013 breakthrough of the year (2). Immunotherapy comes in several forms: immune-modifying agents (antibodies and cytokines); immune cell therapy; therapeutic cancer vaccines; and immune checkpoint inhibitors. Of these, therapeutic cancer vaccines have generally been regarded as having the greatest potential but, despite the tremendous promise and years of research, there is only one FDA-approved cancer vaccine (Dendreon’s Provenge) and dozens of failures. However, research into all types of cancer immunotherapy has been given a new lease of life by the approval of several checkpoint inhibitors in the past five years, and research on cancer vaccines continues.
Cancer vaccines can be divided into four broad categories: peptide, tumor cell, dendritic cell, and DNA (3). Tumor and dendritic cell cancer vaccines can be further subdivided as homologous (derived from the patient) or heterologous (off-the-shelf). Although one might expect that homologous cancer vaccines would possess superior efficacy and safety, off-the-shelf products have the advantage of less labor-intensive preparation. Unfortunately, the discussion is largely academic, as none of these types of cancer vaccine have yielded the amazing improvements in survival that were hoped for.
Nanoparticles to the rescue?
Part of the problem is that the immune system has largely evolved to detect foreign antigens from bacteria, viruses and parasites. Cancer cells are not foreign, but simply normal cells gone awry, which means that the immune system has difficulties recognizing tumor-associated antigens (TAAs), even when presented in the form of a vaccine. By delivering TAAs or DNA encoding TAAs directly to the cells of the immune system, nanoparticles can improve immune presentation and maximize the response. Nanoparticle formulations are already used for preventative vaccines such as Inflexal (liposomal influenza hemagglutinin), Engerix B, Gardasil, and Cervarix (4). Nanoparticles have another big advantage: they are customizable. The nanoparticle composition, surface functionalization, size, and loading strategy can all be fine-tuned to achieve the desired profile, with maximum efficacy and minimum side effects.
It is not enough to induce a strong immune response. For a cancer vaccine to be successful, it has to induce the right type of response. In broad terms, an immune response can occur either via a B cell antibody-mediated response or a cytotoxic T cell-mediated response (5). Immunogenic cancer cell death is primarily achieved by the latter, which therefore needs to be maximized. But to generate a cytotoxic T cell response, antigens have to be processed and presented through major histocompatibility complex (MHC) Class I pathway. Unfortunately, most tumor antigens are presented by MHC Class II pathways. Nanotechnology can help – simply conjugating antigens with nano- and micro-particles can result in a thousand-times increase in antigen presentation via the MHC Class I pathway and can thus ensure a cytotoxic T lymphocyte response. Adding antibodies to the nanoparticle surface allows targeting of specific immune cells, ensuring that exactly the right immune response is activated.
Liposomes and polymer nanoparticles are the most commonly used nanotechnology approaches for cancer vaccines. These particles are frequently used so we have a great deal of experience working with and manipulating them, plus there should be few safety concerns when entering clinical trials.
Nanoparticle composition, size, shape, surface chemistry, and surface charge influence the magnitude and type of immune response. It appears that the size and surface modification have the greatest influence on antigenicity, adjuvanticity, inflammation, and uptake (6). However, there may be trade-offs between the various properties, which must be weighed when designing nanoparticle-based cancer vaccines.
Sizing up immune response
Nanoparticles used for vaccination strategies typically have a size range of 20-200 nm, which is smaller than many other antigens, such as emulsions, mineral salts, and whole cell vaccines. Many peptide antigens are small (<10 nm) and therefore dendritic cells and macrophages do not readily recognize them. The small size and high surface area to volume ratio of nanoparticles allows for a large loading capacity and can increase antigen exposure to dendritic cells. A nanoparticle size of 25-50 nm appears to yield the best results.
Nanoparticle cancer vaccines do not target tumors directly – they target dendritic cells. Dendritic cells, present in peripheral tissues and lymph nodes (7), are the primary antigen-presenting cells and help dictate whether an immune response will be induced and what type. The size of the nanoparticle influences the type of dendritic cells that antigens are presented to. When administered intradermally, larger nanoparticles (100 nm) interact with dermal dendritic cells. These cells must then migrate to the lymph nodes to generate a robust cytotoxic T cell immune response. Smaller nanoparticles (25 nm), however, can go straight to the lymph nodes. The lymph nodes are home to the majority of the body’s dendritic cells, and are the only place where CD8+ dendritic cells are found, which activate cytotoxic T cell responses. The smaller size also translates to a higher uptake by dendritic cells and longer residence in the lymph nodes, resulting in a stronger T-cell based immune response.
So, in general, smaller is better. But there is such a thing as too small. Ultra-small nanoparticles (<10nm) have poorer receptor binding affinities and internalization than relatively larger nanoparticles, possibly due to dissociation of smaller nanoparticles prior to internalization. Ultimately, the size of nanoparticle chosen depends on the response you want to provoke. Immunization with nanoparticles of 40 and 49 nm diameter induced production of interferon-γ and a T cell-mediated response (8), the proposed mechanism of action for cancer vaccines. However, immunization with larger nanoparticles (93-123 nm) was ideal for inducing an antibody-mediated response. Shape also plays a part: spherical nanoparticles favor elicitation of a T cell-mediated response whereas rod-shaped nanoparticles lean towards an antibody-mediated response (Figure1).
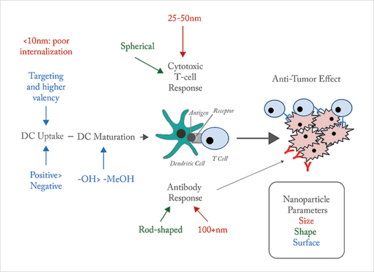
Figure 1. Altering response. DC: dendritic cell. Nanoparticle characteristics affect the performance at different aspects of dendritic cell-mediated immune activation and response.
Scratching the surface
The surface of the nanoparticle is the first thing that dendritic cells interact with. Therefore, it is unsurprising that proper surface modification is a key factor in whether a cancer vaccine will be successful. The first aim is to get the nanoparticle inside the dendritic cells. Positively charged particles tend to be more easily internalized than their negative counterparts, particularly for larger nanoparticles (9). This is primarily driven by electrostatic interactions with the negatively charged cell membrane. A positive charge is especially valuable for DNA vaccines as it will be more effective in condensing the DNA, protecting it from degradation, and traversing the cell membrane. The downside of positively charged nanoparticles is that there is more chance of interaction and uptake by other cells, leading to concerns over toxicity (10).
Another study found that dendritic cell maturation was accelerated when nanoparticles with hydroxyl surface groups were used, compared to those with methoxy surface groups (11). Many tumor antigens are soluble peptides that are weakly immunogenic by themselves; attachment onto a hydrophobic nanoparticle surface can improve their immunogenicity. Furthermore, the way an antigen is attached onto the surface influences the immune response magnitude. Conjugated antigens tend to result in higher T cell responses compared with antigens that are adsorbed onto the nanoparticle surface (12).
Targeting is another advantage of nanoparticles. Attachment of antibodies, peptides, and aptamers can help increase the interaction with and accumulation in a certain type of tissue or cell. Furthermore, the use of a targeting ligand leads to receptor-mediated cell uptake. Dendritic cells recognize antigens using C-type lectin receptors, including mannose receptors. Decorating nanoparticles with mannose has been shown to increase interaction with dendritic cells and a higher valency of mannose ligands improves uptake (13).
Boosting response
Adjuvants – additives to boost immunogenicity – can make or break a successful vaccine. Nanoparticles themselves can act as adjuvants. Traditional adjuvants, such as mineral salts and emulsions, form a depot and slowly release antigens. Nanoparticles can produce a similar effect by encapsulating antigens within a liposome or polymer matrix. The delayed onset of action seen with nanoparticle formulations and co-delivery of antigens with adjuvants or immune-stimulatory chemotherapeutics may result in higher immunogenic cancer cell death.
Cancer vaccines have so far mostly failed to achieve sufficient immune responses in terms of both intensity and duration. Even where vaccines have elicited an immune response, this has not translated to effective tumor killing. Nanotechnology is primed to accelerate the development of this straggling field. The flexibility of nanoparticle design could finally unlock directed immune responses with adequate magnitude.
There’s an important caveat. So far, nanotechnology-based cancer vaccines have been limited to laboratory tests; the real test will be whether nanotechnology-based cancer vaccines result in improved patient outcomes and survival. Given the multifaceted, ever-evolving nature of cancer, and the disappointing results of previous ‘cures’, it may be best not to pin too much hope on a single approach. For the best chance of meaningful survival improvement for patients, we may need to look at a mainstay of cancer treatment – combination therapy. Combining cancer vaccines and checkpoint inhibitors could be an interesting potential therapeutic regimen, with cancer vaccines providing acceleration of the immune response and checkpoint inhibitors ‘releasing the brakes’ on immune system inhibition. When you are dealing with an enemy as deadly and evasive as cancer, a single solution is unlikely to be enough. That said, if nanoparticles can fulfil their promise, we will be adding a powerful new weapon to our armory.
Ronak Savla is an Applied Drug Delivery Fellow with Catalent Pharma Solutions through the Rutgers Pharmaceutical Industry Fellowship Program.
- M. Vanneman and G. Dranoff, “Combining Immunotherapy and Targeted Therapies in Cancer Treatment”, Nat. Rev. Cancer 12(4), 237-251 (2012).
- J. Couzin-Frankel, “Cancer Immunotherapy”, Science; 342(6165), 1432-1433 (2013).
- C. Witten, “U.S. FDA and Cancer Vaccine Development” ,US Food and Drug Administration: Center for Biologics Evaluation and Research, (2 June 2008), www.fda.gov
- M. F. Bachmann and G. T. Jennings, “Vaccine Delivery: A Matter of Size, Geometry, Kinetics and Molecular Patterns”, Nat. Rev. Immunol. 10(11), 787-796 (2010).
- J. M. Silva et al., “Immune System Targeting By Biodegradable Nanoparticles For Cancer Vaccines”, J. Control. Release 168(2), 179-199 (2013).
- M. Zaman, M. F. Good and I. Toth, “Nanovaccines and Their Mode of Action”, Methods 60(3), 226-231 (2013).
- S. T. Reddy et al., “Exploiting Lymphatic Transport and Complement Activation in Nanoparticle Vaccines”, Nature Biotechnol. 25(10), 1159-1164 (2007).
- P. L. Mottram et al., “Type 1 and 2 Immunity Following Vaccination is Influenced by Nanoparticle Size: Formulation of a Model Vaccine for Respiratory Syncytial Virus”, Mol. Pharm. 4(1), 73-84 (2007).
- C. Foged et al., “Particle Size and Surface Charge Affect Particle Uptake by Human Dendritic Cells in an In Vitro Model”, Int. J. Pharm. 298(2), 315-322 (2005).
- V. Shah V et al., “Genotoxicity of Different Nanocarriers: Possible Modifications for the Delivery of Nucleic Acids”, Curr. Drug Discov. Technol. 10(1), 8-15 (2013).
- R. B. Sim et al., “The Covalent-Binding Reaction of Complement Component C3”, Biochem. J. 193(1), 115-127 (1981).
- L. Zhao et al., “Nanoparticle Vaccines”, Vaccine 32(3), 327-337 (2014).
- S. Espuelas et al., “Influence of Ligand Valency on the Targeting of Immature Human Dendritic Cells by Mannosylated Liposomes”, Bioconjug. Chem. 19(12), 2385-2393 (2008).
“How many people can truly say that they are working on what they dreamed of as kids? I guess I’m one of the lucky ones.” After learning about the field of nanotechnology as a high school student, Ronak saw the opportunity of combining his interest in science with his passion to help others. Ronak started working in a nanotechnology drug delivery lab during the summer after his freshman year at Rutgers University. He continued developing innovative nanoparticle drug delivery solutions during pharmacy school and graduate school. This has launched him to his current position as the Applied Drug Delivery Fellows at Catalent Pharma Solutions as part of the Rutgers Pharmaceutical Industry Fellowship Program.