Going Native with Microfluidics
Microfluidics technology has room for improvement, but can undoubtedly impact protein analysis.
Andrew Lynn | | Opinion
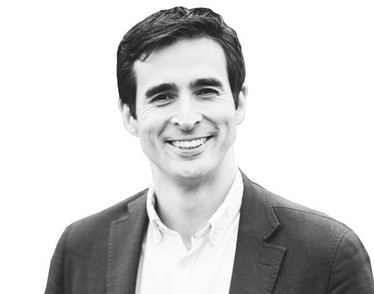
When it comes to assessing the key characteristics of complex protein-based biotherapeutics, one of the main challenges is analyzing in native conditions. It is important to ensure that the protein is in native or near-native conditions, in solution, with relevant excipients, and that it remains this way throughout the analysis. You also need to ensure that only the protein portion is read, but when you have a complex solution with excipients and additives present, these can interfere with the read.
But we should also acknowledge that proteins don’t always function alone – the interactions of a protein can be instrumental. We need to consider proteins not as isolated entities, but as part of rich, complex networks that ultimately drive biological systems.
In my view, techniques that rely on surface interactions or denaturation just can’t give us a true picture of the protein, but in my microfluidics technology can. At the microfluidic scale turbulence can be readily avoided and so fluid behaviour is more predictable than microfluidic flow. This in turn implies improved reliability and reproducibility of experiments. Importantly, with well-defined flow it is possible to perform experiments in solution instead of surface-bound. Testing in solution is of course relevant for basic metrics like size, but it becomes even more vital as research shifts to looking at interactions. Often the interactions of a protein with other molecules is key, so being able to observe these without compromise or risk of artefacts is crucial.
Most of the unique and functional characteristics of proteins come down to their conformation. Analyses where the molecules interact with a surface or matrix, or are fixed to a surface, can affect that unique conformation, or ensemble of conformations, meaning that what you’re testing is not representative of the native solution-state protein. The dominant techniques for measuring protein-protein interactions, such as surface plasmon resonance (SPR) or bio-layer interferometry (BLI), rely upon fixing a protein to a surface to detect binding to an interaction partner. But these techniques come with an inherent risk that the experiment is impacted by the surface coupling – and the question of whether an observation is an accurate representation of the biological interaction. By testing with a microfluidic system in solution, you can more closely mimic real biological conditions.
As a field, microfluidics is still growing, particularly in its application to analysis of proteins. Current systems are limited in what they can analyse; for example we might only be able to separate a mixture based on one characteristic. Over time, I expect to see this changing, with microfluidic systems being used for more complex manipulations or separations that allow for more in-depth insights.
Using microfluidics can be challenging but that the benefits offered by the technology makes it worth persisting. It is useful to consider the modular nature of microfluidic systems, and if a particular chip is proving difficult to operate, try to break the chip down into unit operations and then troubleshoot each of them individually before reassembling the complete design.
Right now, there are relatively few off-the-shelf microfluidic instruments so most microfluidic research takes place on home-built rigs with custom chips, making it the domain of the expert. This means that groups using microfluidics need specialist team members and equipment. While viewed as generally beneficial due to the reduced sample consumption, the small volumes and path-lengths used in microfluidic devices can create challenges around detection sensitivity. Reproducibility is often a key issue – when you’re looking at things with such great sensitivity, small changes are magnified and this can result in increased variability of the output results.
That’s why there’s a movement now to get away from bespoke handcrafted rigs to standardized instruments – these allow researchers to harness all the benefits of microfluidics, without having to get lost in worrying about reproducibility.
I am particularly interested in microfluidic diffusional sizing (MDS), which came out of the lab of Professor Tuomas Knowles at the University of Cambridge. This technology should allow for the native state analysis of proteins and protein complexes with high accuracy down to low concentrations. The original findings were published by Yates et al in Nature Chemistry, 2015 – but this work was all performed on a handcrafted rig in the lab! Now the aim is to develop a “plug and play” system for MDS – and these are starting to emerge.
In the future, I also hope that new systems will allow us to look at proteins and antibodies binding and interacting with a host of other molecules, so that we can further improve our understanding in this area and perhaps even change the way we think about proteins altogether. Developing new and orthogonal approaches to on-chip separation of complex mixtures would be a powerful addition to the microfluidic toolkit and would allow ever more sophisticated experiments to be carried out on-chip.
If researchers have tools to look beyond individual proteins – beyond even individual interactions – and can observe the rich networks of the proteome, where interactions between proteins, or between proteins and other molecules occur, they could develop deep, system-wide understanding of biological complexity. This would improve our ability to comprehend the full impact of perturbing these biological systems and allow the development of more selective treatments.
Microfluidic techniques allow us to look at these interactions in solution, and that is where real leaps in our understanding of biology will be made.